CPB for neonates, infants and children differs from CPB for adults in various respects: dimensions, haemodilution, metabolic requirements, immature physiological regulation mechanisms, specific characteristics of heart disease, pH regulation, inflammation intensity, cerebral perfusion, and the occasional need to perform deep hypothermic circulatory arrest [10,12,44].
Dimensions
The most obvious issue is the child's size in relation to equipment [6,12,44].
Dimensions
The most obvious issue is the child's size in relation to equipment [6,12,44].
- Children’s vena cavae are smaller and easily obstructed by cannulas – risk of superior vena cava syndrome, brain oedema, liver stasis, and ascites. If venous pressure (Pv) rises, perfusion pressure during CPB (Partery - Pvein) may be locally insufficient if mean arterial pressure (MAP) does not rise, hence a risk of cerebral or splanchnic ischaemia. Venous drainage into the reservoir may be facilitated by aspiration (pump or vacuum at - 30 mmHg); this allows the use of smaller venous canulae..
- The aortic cannula must be appropriately sized to reduce the risk of obstruction and limit the haemolysis rate at the maximum possible flow rate (3.2 L/min/m2).
- The smaller the circuit, the larger the foreign surface area in contact with the blood in relation to volume, and therefore the more intense the mechanical lesions of the blood cells and the release of vasoactive substances.
- The CPB volume (priming) volume represents 50-100% of the child’s circulating volume, compared to a ratio of 0.25:1 in adults. The priming volume is 200 - 400 mL compared to an infant’s circulating volume of 200-500 mL. For children weighing < 5 kg, circuits are available with a priming volume of 180-250 mL (Capiox FX™, Lilliput™). Some systems’ oxygenators are placed on the operating table and the volume is limited to 120-150 mL. For children weighing 5-10 kg, priming varies from 270 to 375 mL. Priming and haemodilution may be reduced by gradually draining the patient's blood into the CPB circuit before activating it (retro-priming) and draining the clear liquid into a transfer bag. The child's arterial pressure is maintained with a vasoconstrictor.
- The intensity of the inflammatory response can be reduced by a biocompatible coating of the plastic tubings with heparin or poly-methoxyethyl-acrylate, or by the deposit of albumin from the priming solution [29].
Due to their small size, children's surface area is high compared to their weight, which, combined with their immature thermoregulation systems, increases heat exchange with their external environment and increases the risk of heat loss in the operating theatre. General CPB technique is covered in Chapter 7 (see Machines and Circuits).
Haemodilution
The pump's high priming volume imposes a considerable dilution to the circulating volume of children (80 mL/kg from 10-20 kg, 70 mL/kg > 20 kg), and even more to infants' blood volume (85 mL/kg < 10 kg) [44]. This haemodilution reduces oncotic pressure and haematocrit, which increases tissue oedema and reduces peripheral arterial resistance. It reduces platelet concentration and coagulation factors and lowers the serum blood levels of circulating drugs. The risk of sudden recovery and decurarisation on activation of CPB is very high in young children. This explains the recommendation of “anaesthetising” the pump prior to initiating CPB by adding induction-dose opiates and curare to the priming liquid.
Low-temperature haemodilution is required to prevent an excessive increase in viscosity, which would slow the flow rate in the microcirculation (sludging). Viscosity is generally stable if the haematocrit value (% Ht) is the same as the temperature value (T°C). The required degree of haemodilution equates to 20% haematocrit at a temperature of 18°C and 25% at a temperature of 28°C. These values improve the blood's rheological characteristics by reducing its viscosity without compromising the oxygen delivery (DO2) required for meeting metabolic requirements at hypothermia. 18% Ht is tolerated without any acidosis at low temperatures, although there is a risk of more severe neurological sequelae [25]. Indeed, low Ht is clearly linked to brain damage and subsequent neurological sequelae. It is therefore recommended that the level is not allowed to drop below 24% under deep hypothermia and below 25-30% under moderate hypothermia [19,22]. Whole blood with a shelf life of less than 48 hours (less than 6 hours for neonates) is added to the fluid and electrolyte priming liquid to achieve Ht of 24-28%.
Target Ht during CPB (HtCPB) after dilution by the priming volume (PVCPB) is calculated as follows (BV: blood volume, Htpreop: child's Ht prior to operation):
Haemodilution
The pump's high priming volume imposes a considerable dilution to the circulating volume of children (80 mL/kg from 10-20 kg, 70 mL/kg > 20 kg), and even more to infants' blood volume (85 mL/kg < 10 kg) [44]. This haemodilution reduces oncotic pressure and haematocrit, which increases tissue oedema and reduces peripheral arterial resistance. It reduces platelet concentration and coagulation factors and lowers the serum blood levels of circulating drugs. The risk of sudden recovery and decurarisation on activation of CPB is very high in young children. This explains the recommendation of “anaesthetising” the pump prior to initiating CPB by adding induction-dose opiates and curare to the priming liquid.
Low-temperature haemodilution is required to prevent an excessive increase in viscosity, which would slow the flow rate in the microcirculation (sludging). Viscosity is generally stable if the haematocrit value (% Ht) is the same as the temperature value (T°C). The required degree of haemodilution equates to 20% haematocrit at a temperature of 18°C and 25% at a temperature of 28°C. These values improve the blood's rheological characteristics by reducing its viscosity without compromising the oxygen delivery (DO2) required for meeting metabolic requirements at hypothermia. 18% Ht is tolerated without any acidosis at low temperatures, although there is a risk of more severe neurological sequelae [25]. Indeed, low Ht is clearly linked to brain damage and subsequent neurological sequelae. It is therefore recommended that the level is not allowed to drop below 24% under deep hypothermia and below 25-30% under moderate hypothermia [19,22]. Whole blood with a shelf life of less than 48 hours (less than 6 hours for neonates) is added to the fluid and electrolyte priming liquid to achieve Ht of 24-28%.
Target Ht during CPB (HtCPB) after dilution by the priming volume (PVCPB) is calculated as follows (BV: blood volume, Htpreop: child's Ht prior to operation):
HtCPB = (Htpreop • BVchild) / (PVCPB + BVchild)
Supplementary blood (BVsuppl) is calculated simply as follows (RCV: red cell volume):
BVsuppl = RCVchild - RCVCPB
where: RCVchild = BVchild • Htpreop
RCVCPB = (BVchild + PVCPB) • HtCPB
The priming liquid is a low-calcium buffered fluid and electrolyte solution (Ringer-lactate, Plasma-lyte A). Albumin (5% up to 25%) or fresh frozen plasma (FFP) are added to maintain oncotic pressure at around 18 mmHg and limit interstitial exudate [15]. Albumin tends also to decrease the transfusion rate (red blood cells and platelets) and the postoperative weight gain, but it has no impact on mortality. Albumin can be replaced by fresh frozen plasma [29]. Some centres also add mannitol to ensure diuresis and act as a neutraliser of free radicals, and a steroid to minimise inflammatory response. The whole blood and steroids tend to induce hyperglycaemia. In children aged < 4 months, blood is usually added to the priming fluid or during CPB to maintain sufficient Ht (> 24%) [47]. When possible, fresh (< 72 hours) leukoreduced, irradiated, CMV-negative blood is used, since the potassium and lactate levels in older allologous bags are excessive; moreover, citrate causes hypocalcaemia [6,12]. Adding calcium to the priming solution decreases the degree of hypocalcemia induced by haemodilution and transfused blood, but without impact on morbidity and mortality [29].
Ultrafiltration is commonly used with children to limit haemodilution, impede inflammatory response, and improve fluid and electrolyte balance, gas exchange, or multiple organ performance. It is performed during CPB (CUF: conventional ultrafiltration) and once the patient is off bypass (MUF: modified ultrafiltration) (see Chapter 7 Haemofiltration) [39]. The aim of MUF is to remove fluid (750 mL/m2) or achieve a specific Ht (40%) [44].
High metabolic requirements
Infants’ high metabolic requirements (100-150 kcal/kg/24h, VO2 6-8 mL/kg/min) are met by a 30-50% higher cardiac output than that observed in adults. At normothermia, the CPB flow rate is generally 100-150 mL/kg/min for young children, but may be as high as 150-200 mL/kg/min for premature infants (< 3 kg). The older the child is, the closer the rate is to that applied to adults (50 mL/kg/min) [10,12,24].
- < 2.5 kg 175 mL/kg/min 3.2 L/min/m2
- 2.5-5 kg 150 mL/kg/min 3.0 L/min/m2
- 5-15 kg 125 mL/kg/min 2.8 L/min/m2
- 15-30 kg 100 mL/kg/min 2.6 L/min/m2
- 30-50 kg 75 mL/kg/min 2.5 L/min/m2
- > 50 kg 50 mL/kg/min 2.4 L/min/m2
In hypothermia (< 25°C), the flow rate may be reduced to 80 mL/kg/min for children weighing < 10 kg. By measuring SvO2 on the CPB venous return, it is possible to determine whether perfusion is meeting the body's requirements. A higher flow rate through smaller cannulas increases the risk of haemolysis compared to the situation for adults. Although pulsatile perfusion may be a more effective means of improving myocardial, renal and pulmonary performance for children than for adults, it presents a greater risk of haemolysis during systolic acceleration of the flow through a small arterial cannula [2].
Arterial pressure is low in children. At birth, it is 65/45 mmHg and at 1 year, it is 90/45 mmHg. A mean perfusion pressure of 40-45 mmHg is usually sufficient at normothermia. This falls to 30-40 mmHg in hypothermia (≤ 28°C). The heart of children with cyanosis or congestive failure is less tolerant to ischaemia than the heart of normal neonates, while the latter appear to be more resistant than the heart of adults.
Immature physiological regulation
Infants’ functional immaturity affects all the organs [4,10,26].
Arterial pressure is low in children. At birth, it is 65/45 mmHg and at 1 year, it is 90/45 mmHg. A mean perfusion pressure of 40-45 mmHg is usually sufficient at normothermia. This falls to 30-40 mmHg in hypothermia (≤ 28°C). The heart of children with cyanosis or congestive failure is less tolerant to ischaemia than the heart of normal neonates, while the latter appear to be more resistant than the heart of adults.
Immature physiological regulation
Infants’ functional immaturity affects all the organs [4,10,26].
- Low cardiac contractility, fixed and heart-rate-dependent stroke volume;
- Low pulmonary compliance, low functional residual capacity, and closing volume attained at tidal volume – loss of surfactant and interstitial oedema during CPB;
- Fragile capillary membranes and heightened risk of capillary leak syndrome;
- Kidneys incapable of concentrating urine normally and excreting water and Na+ – the incidence of acute renal failure is 3-8% following infant cardiac surgery;
- Coagulopathy: thrombocytopaenia, platelet dysfunction, low hepatic synthesis of coagulation factors;
- Under stress, return to transitional haemodynamic conditions (see Figure 14.4).
Cyanotic children present with platelet dysfunction and are deficient in factors II, V, VII, VIII, IX and fibrinogen [10,35]. Platelet transfusions (1 U per 10 kg of weight) are generally required. The systemic inflammatory reaction is very severe after CPB with exacerbation of interstitial leakage and multiple organ failure requiring haemofiltration. These characteristics are countered by the greater resistance to ischaemia and hypothermia of normal neonate organs. However, this observation does not apply to the heart, since the immature myocardium is more sensitive to stress than the adult myocardium [6]. Hypoglycaemia is a major risk in neonates since their gluconeogenesis is still deficient. Blood glucose levels should be carefully monitored throughout the operation and corrected if required by a 20-30% dextrose infusion.
Specific features of heart disease
If aortopulmonary shunting is present (patent ductus arteriosus, aortopulmonary collaterals, Blalock-Taussig surgical shunts), a considerable volume of blood is diverted from the systemic circulation at the beginning of CPB. If the pulmonary circulation is bypassed and its normal pressure levels plummet, blood continues to flow through these conduits, gradually filling the left atrium and ventricle and causing pulmonary venous reflux with a risk of pulmonary oedema. The operator must occlude these shunts before activating the pump and drain the ventricle (venting) during CPB to prevent three problems.
Specific features of heart disease
If aortopulmonary shunting is present (patent ductus arteriosus, aortopulmonary collaterals, Blalock-Taussig surgical shunts), a considerable volume of blood is diverted from the systemic circulation at the beginning of CPB. If the pulmonary circulation is bypassed and its normal pressure levels plummet, blood continues to flow through these conduits, gradually filling the left atrium and ventricle and causing pulmonary venous reflux with a risk of pulmonary oedema. The operator must occlude these shunts before activating the pump and drain the ventricle (venting) during CPB to prevent three problems.
- Excessively low systemic pressure despite the high pump flow rate – the shunt effect is exacerbated by administering a vasoconstrictor.
- Prolonged systemic (and particularly cerebral) cooling time due to the diversion of blood flow by pulmonary recirculation.
- The operating field is flooded by the reflux if the left chambers are open – these chambers are dangerously distended if closed.
If present, the persistent left superior vena cava (LSVC) drains into the coronary sinus – in this case, retrograde cardioplegia is impossible, since the infusate leaks into the systemic vascular bed.
Anticoagulation
Infants aged under one year differ significantly from older children and adults in terms of CPB anticoagulation [14].
Anticoagulation
Infants aged under one year differ significantly from older children and adults in terms of CPB anticoagulation [14].
- Their coagulation system is immature with levels of many factors 30-70% lower.
- Congenital heart diseases are combined with platelet hypoaggregability and a shortage of von Willebrand factor – hypoxaemia prompted by cyanotic disorders results in a reduction in the number of platelets and under-production of vitamin K-dependent clotting factors.
- Haemodilution is more severe (40-60% increase in circulating volume);
- Levels of antithrombin III are low, which limits heparin anti-Xa activity; therefore its dosage must be increased (400 IU/kg instead of 300 IU/kg) [17]. In the event of increased heparin resistance (> 600 IU/kg and ACT < 450 secs), an AT III deficit should be strongly suspected and must be replenished [27].
- In our experience (CHUV), a dose of 25 IU/kg (max 100 IU/kg) of AT III (Kybernin®, Atenativ®) in the CPB, repeated once if required, is sufficient to correct this deficit and enables an appropriate ACT to be achieved (> 450 sec). FFP may also be added, but the required volume is much higher than with a concentrated preparation.
To minimise the effects of CPB on this delicate system, biocompatible circuits imitating the properties of the endothelium are given preference. Regardless of the materials used and the circuit size, anticoagulation is performed with unfractionated heparin (UFH 4 mg/kg) in order to reach an ACT > 480 seconds, although ACT tends to provide an overestimation of the actual degree of anticoagulation in young children [17]. This dose, which is administered prior to cannulation (by central route or directly in the RA) is immediately diluted by the priming volume on initiation of CPB, and therefore a dose of UFH must be added to the CPB circuit. Modified ultrafiltration performed at the end of CPB removes water, electrolytes and small molecules – heparin is concentrated and its levels rise as its molecule is too large to be filtered.
Once the results of the operation are considered satisfactory and a second run of CPB is unlikely, protamine is administered in a 1:1 ratio with UFH or based on the child’s weight (3-4 mg/kg) [44]. If the equipment is available, a quantitative measurement of circulating heparin (Hepcon™) is more appropriate. The incidence of hypotensive reactions to protamine is lower in children than in adults (1.7-2.9%) [37].
Antifibrinolytics (tranexamic acid and ε-aminocaproic acid) offer the same efficacy in children as in adults. The recommended dosages for tranexamic acid are as follows [43].
Once the results of the operation are considered satisfactory and a second run of CPB is unlikely, protamine is administered in a 1:1 ratio with UFH or based on the child’s weight (3-4 mg/kg) [44]. If the equipment is available, a quantitative measurement of circulating heparin (Hepcon™) is more appropriate. The incidence of hypotensive reactions to protamine is lower in children than in adults (1.7-2.9%) [37].
Antifibrinolytics (tranexamic acid and ε-aminocaproic acid) offer the same efficacy in children as in adults. The recommended dosages for tranexamic acid are as follows [43].
- < 2 months: 50 mg/kg, 7 mg/kg/h infusion;
- 2-12 months: 25 mg/kg, 6 mg/kg/h infusion;
- > 12 months: 15 mg/kg, 5 mg/kg/h infusion.
pH regulation
At low temperatures, the water dissociation constant and the solubility of gases in liquids increase. As such, the value of PaCO2 measured in a normal blood sample (PaCO2 = 40 mmHg) cooled to 27° is only 23 mmHg, even though no exchange has occurred with the external environment, because the soluble fraction of the gas [HCO3] has increased. At 20°, the normal apparent pH is 7.7 and PaCO2 is 18 mmHg. Acid-base regulation can be achieved clinically by two techniques (see Figure 14.23).
At low temperatures, the water dissociation constant and the solubility of gases in liquids increase. As such, the value of PaCO2 measured in a normal blood sample (PaCO2 = 40 mmHg) cooled to 27° is only 23 mmHg, even though no exchange has occurred with the external environment, because the soluble fraction of the gas [HCO3] has increased. At 20°, the normal apparent pH is 7.7 and PaCO2 is 18 mmHg. Acid-base regulation can be achieved clinically by two techniques (see Figure 14.23).
- In alpha-stat mode, total CO2 content is kept constant, but readings are performed using a device with a sample adjusted to 37°, as if the patient were normothermic. Since gas solubility is increased at low temperatures, the partial pressure is actually lower; the blood becomes artificially alkaline and hypocapnic, but the [H+]/[OH-] ratio remains constant. In such situations, cerebral autoregulation is unimpaired, extracellular acidosis is reduced, pHi is kept stable, and intracellular enzyme functions are fully preserved [42].
- In pH-stat mode, the blood's pH level is kept at 7.4 irrespective of temperature by adding CO2 to the ventilated gas (3-5%; CO2 content rises (apparent hypercapnia) and pH falls. If pH is kept at 7.4 at 17°, the sample read at 37° gives a value of 7.08 (equivalent PaCO2: 110 mmHg). The oxygen-haemoglobin dissociation curve is displaced to the right. This technique prompts hypercapnic cerebral vasodilation; autoregulation is lost and the luxuriant flow becomes pressure-dependent [31]. Despite the extra-cellular acidosis, the intracellular pH is only slightly modified [38].
Figure 14.23: Regulation of acid-base balance in hypothermia. In alpha-stat mode, total CO2 content is kept constant, but readings are performed using a device with a sample adjusted to 37°, as if the patient were normothermic. Since gas solubility is increased at low temperatures, the partial pressure is actually lower; the blood becomes artificially alkaline and hypocapnic, but the [H+]/[OH-] ratio remains constant. In pH-stat mode, the blood pH level is kept at 7.4 irrespective of temperature by adding CO2 to the ventilated gas; CO2 content rises (apparent hypercapnia) and pH falls. If pH is kept at 7.4 at 17°, the sample read at 37° gives a value of 7.08 (equivalent PaCO2: 110 mmHg).
The alpha-stat technique is normally routinely used for CPB with moderate hypothermia (25-30°C) in adults, since these conditions offer significantly superior neurological results at 6 weeks and 2 months [33]. The situation appears to be different for young children since the pH-stat strategy offers the benefit of doubling cerebral blood flow (CBF), since CO2 is a powerful cerebral vasodilator. Since the homogeneity of heat exchange is improved, the pH-stat technique is indicated during cooling and rewarming as it favours fast, uniform thermal variations of the brain, notably during full circulatory arrests at < 20°C (Figure 14.24) [20,21].
Figure 14.24: Increased cerebral blood flow (CBF) during pH-stat compared to alpha-stat regulation in different areas of the brain: hemispheres, basal ganglia, midbrain and brainstem. It is evident that all structures are affected. HT: after 30 minutes of hypothermia. R5: after 5 minutes of rewarming [3].
In children, immediate neurological results tend to be superior in pH-stat after deep hypothermic circulatory arrest [3,13,48], although mental development at 4 years is no different [5]. The difference between adults and children probably lies in the fact that in adults, neurological sequelae are primarily due to emboli, whereas in children, lesions tend to be secondary to global ischaemia. Vasodilation prompted by the pH-stat technique increases risk in the former case and reduces it in the latter.
Proteins act as weak acids at the usual pH of blood and are a major buffer system for acid-base balance. Unlike the bicarbonate system, their buffering power is unimpaired at low temperatures, since the degree of dissociation from the imidazole group of histidine does not change according to temperature. Therefore, under hypothermia, acidosis should be corrected by adding proteins since the buffering power of bicarbonate is rendered ineffective < 28°C.
Systemic inflammatory response syndrome (SIRS)
The smaller the child, the more intense the inflammatory reaction, since the foreign surface area (CPB circuit, aspirators, filters) and triggers (temperature variations, low flow) are proportionately greater than in adults. Moreover, neurohumoral balance and target cells are more fragile in infants. When present, pulmonary hypertension exacerbates inflammatory lesions in the lungs.
Endotoxins, complement, kallikrein and cytokines are the humoral elements of the reaction – these mediators activate the neutrophils and endothelial cells. Binding of leukocytes to these cells marks the initial stage of the inflammatory reaction. It is triggered by the expression of specific molecules on the surface of both types of cells. The leukocytes can then migrate in the extravascular space where they release their toxins (proteases and free radicals), which damage the surrounding tissue. Mechanical lesions related to the pump, oxygenator and filters, and contact between the blood and foreign surface area (circuits) and with the air (aspiration, venous reservoir) are the main triggering factors. Over 50% of neutrophils are sequestrated in the lungs during rewarming – their degranulation contributes to pulmonary cellular damage. CPB duration, hypothermic depth and degree of haemodilution are aggravating factors [40].
Numerous tracks have been explored in the hope of reducing the intensity of this reaction. The most commonly used solutions are as follows [41].
- Use of heparinised circuits – reduces platelet adhesiveness and adsorption of coagulation factors, alleviates pulmonary, renal and neurological complications [26].
- Circuits rendered biocompatible by treatment with polymers (poly(2‐methoxyethyl acrylate)) or anti-inflammatory molecules (factor H inhibiting C3a complement) – impedes complement cascade and leukocyte activation [36].
- Haemofiltration during CPB (rewarming) – in children, this has a beneficial effect by eliminating fluid excess due to haemodilution and improving gas exchange. It eliminates a large proportion of cytokines, although this also includes those with an anti-inflammatory effect [7]. Haemofiltration offers guaranteed benefits in terms of addressing postoperative complications (reduction of interstitial fluid and multi-organ failure, improvement of ventricular function and haemostasis) [23].
- Drug prophylaxis:
- Digestive decontamination by preoperative antibiotic therapy;
- Administration of steroids – methylprednisolone (10-30 mg/kg) and dexamethasone (1-6 mg/kg) adjust inflammatory response and improve postoperative clinical status according to some studies (see below). Many centres use them routinely in paediatrics [18];
- Anticytokine and monoclonal antibodies (rarely used).
- Modified ultrafiltration – post-CPB, an ultrafiltration system is supplied with blood from the arterial cannula. The blood is returned to the patient via the venous cannula. The circuit is operated by a CPB pump at a rate of 10-30 mL/kg/min for 20-30 minutes. The system is able to ultrafilter 100-150 mL/min and offers four major benefits (Figure 14.25) [1,32]:
- Total water is reduced and haematocrit is increased;
- Blood coagulation is improved;
- Many inflammatory triggers are eliminated;
- SIRS-related failures are reduced and postoperative cardiac, neurological, renal and pulmonary function is improved;
- The only problem is prolonged CPB, which is often irritating for surgeons.
- Cytokine absorber: use of an extracorporeal cytokine absorber (Cytosorb®) in tandem with haemodiafiltration has been described in adults in cases of septic shock and multiple organ failure. Current research is examining its application during CPB in adults. However, an appropriate device for lightweight children is not yet available. Its use in paediatrics is anecdotal at present [8].
Although highly controversial in cardiac surgery (see Chapter 24 - Introduction), use of steroids appears to be effective in high-risk infants (Aristotle score > 10). They reduce postoperative bleeding and inflammatory markers; they shorten the duration of ventilation and intensive care length of stay without increasing the risk of hyperglycaemia or infection [9,18]. They may reduce mortality [45]. Given their onset of action > 45 minutes, steroids should be administered prior to CPB. Indeed, their efficacy is improved if they are administered at induction rather than in the CPB priming solution [9]. The protocol adopted by Necker Hospital in Paris combines both options:
- Dexamethasone (1 mg/kg) in induction for neonates;
- Methylprednisolone (30 mg/kg) in the CPB priming solution for deep hypothermic operations with or without circulatory arrest (Norwood, Sano, Damus-Kaye, total anomalous pulmonary venous return, interruption of the aortic arch).
Nevertheless, the efficacy of steroids for young children is disputed as it is for adults, where their contribution to a reduction in morbidity and morrtality is negligible [11,16,46].
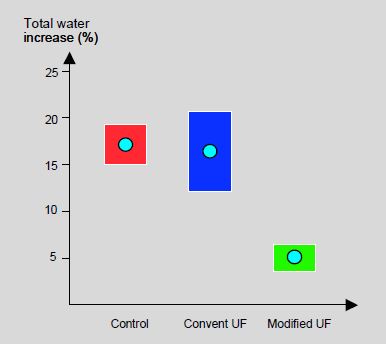
Figure 14.25: Fluid accumulation (total water) without ultrafiltration (control), with conventional ultrafiltration (UF) and with modified ultrafiltration (MUF) post-CPB in children [32]. MUF is significantly more effective. The rectangles represent standard deviations.
Cardioplegia
Although neonates’ myocardium generally withstands ischaemia and reperfusion more effectively than that of adults, cyanosis is associated with reduced myocardial resistance to various stress factors [28]. Maintaining cardiac hypothermia may be difficult if collateral flows are present in cyanotic subjects or in the event of abnormal anatomy of the coronary tree. At low temperatures, there appears to be no difference between crystalloid solutions and blood cardioplegia [49], whereas a blood solution is preferable for hot-shot cardioplegia. A lower K+ concentration is sufficient for achieving cardiac arrest in children. The addition of Ca2+ is beneficial for preserving neonatal myocardium [34].
Cannulations
The size of cannulas should obviously be adjusted to fit vessel size (1/8, 3/16,1/4). In most cases, venous cannulation is performed with two right-angle cannulas inserted in the inferior vena cava and superior vena cava. A single right atrial cannula may be used in the event of aortic valvular or subvalvular lesions, surgery on the great arteries, extracardiac Fontan procedures, Norwood procedures, or anomalous pulmonary venous return [6,35]. The arterial cannula is inserted in the ascending aorta, except in the Norwood procedure (where it is inserted in the ductus arteriosus) or in the event of interruption of the aortic arch. Femoral cannulation is not possible in young children.
Complications
Of the many complications that may occur during CPB (obstructed flow, oxygenator failure, circuit thrombosis, aortic dissection on the arterial cannula, etc.) (see Chapter 7 - Incidents and Accidents), one of the most devastating examples is massive air embolism, since congenital shunting between the systemic and pulmonary circulation results in catastrophic cerebral embolisation [30]. This must be treated immediately, which involves all concerned parties [44].
Figure 14.25: Fluid accumulation (total water) without ultrafiltration (control), with conventional ultrafiltration (UF) and with modified ultrafiltration (MUF) post-CPB in children [32]. MUF is significantly more effective. The rectangles represent standard deviations.
Cardioplegia
Although neonates’ myocardium generally withstands ischaemia and reperfusion more effectively than that of adults, cyanosis is associated with reduced myocardial resistance to various stress factors [28]. Maintaining cardiac hypothermia may be difficult if collateral flows are present in cyanotic subjects or in the event of abnormal anatomy of the coronary tree. At low temperatures, there appears to be no difference between crystalloid solutions and blood cardioplegia [49], whereas a blood solution is preferable for hot-shot cardioplegia. A lower K+ concentration is sufficient for achieving cardiac arrest in children. The addition of Ca2+ is beneficial for preserving neonatal myocardium [34].
Cannulations
The size of cannulas should obviously be adjusted to fit vessel size (1/8, 3/16,1/4). In most cases, venous cannulation is performed with two right-angle cannulas inserted in the inferior vena cava and superior vena cava. A single right atrial cannula may be used in the event of aortic valvular or subvalvular lesions, surgery on the great arteries, extracardiac Fontan procedures, Norwood procedures, or anomalous pulmonary venous return [6,35]. The arterial cannula is inserted in the ascending aorta, except in the Norwood procedure (where it is inserted in the ductus arteriosus) or in the event of interruption of the aortic arch. Femoral cannulation is not possible in young children.
Complications
Of the many complications that may occur during CPB (obstructed flow, oxygenator failure, circuit thrombosis, aortic dissection on the arterial cannula, etc.) (see Chapter 7 - Incidents and Accidents), one of the most devastating examples is massive air embolism, since congenital shunting between the systemic and pulmonary circulation results in catastrophic cerebral embolisation [30]. This must be treated immediately, which involves all concerned parties [44].
- Surgeon: clamping of the aorta, removal of the arterial cannula and cannulation of the superior vena cava (retrograde cerebral flow of 1-2 L/min to remove air) – drainage of the aorta and recannulation.
- Perfusionist: discontinuation of CPB, arterial and venous clamping, drainage of the circuit, and refilling – resumption of CPB at 22-25°C.
- Anaesthetist: patient in forced Trendelenburg position, carotid compression, ventilation at FiO2 1.0, cooling.
CPB in children |
Priming volume close to or greater than the child's circulating volume
CPB flow rate at normothermia: - < 2.5 kg 175 mL/kg/min - 2.5-5.0 kg 150 mL/kg/min - 5-15 kg 125 mL/kg/min - 15-30 kg 100 mL/kg/min - 30-50 kg 75 mL/kg/min - > 50 kg 50 mL/kg/min Perfusion pressure 40-60 mmHg pH regulation in α-stat mode during moderate hypothermia and temperature plateau (> 28°C), but pH-stat when cooling to low temperature (<28°C) and rewarming. Reduction of systemic inflammatory response: heparinised circuits, biocompatible materials, ultrafiltration Routine modified haemofiltration on withdrawal from CPB to impede inflammatory response and reduce fluid excess |
© BETTEX D, BOEGLI Y, CHASSOT PG, June 2008, last update February 2020
References
- AGGARWAL NK, DAS SN, SHARMA G, et al. Efficacy of combined modified and conventional ultrafiltration during cardiac surgery in children. Ann Card Anaesth 2007 ; 10 :27-33
- ALKAN T, AKCEVIN A, UNDAR A, et al. Effects of pulsatile and nonpulsatile perfusion on vital organ recovery in pediatric heart surgery; a pilot clinical study. ASAIO J 2007; 52:530-5
- AOKI M, NORMURA F, STROMSKI ME, et al. Effects of pH on brain energetics after hypothermic circulatory arrest. Ann Thorac Surg 1993; 55:1093-1103
- BAUM VC, PALMISANO BW. The immature heart and anesthesia. Anesthesiology 1997; 87:1529-48
- BELLINGER DC, WYPIJ D, DU PLESSIS AJ, et al. Developmental and neurologic effects of alpha-stat versus pH-stat strategies for deep hypothermic cardiopulmonary bypass in infants. J Thorac Cardiovasc Surg 2001; 121:374-83
- CHARRETTE KA, DAVIES RR, CHEN JM, et al. Pediatric perfusion techniques for complex congenital cardiac surgery. In : MONGERO LB, BECK JR. On bypass. Advanced perfusion techniques. Totowa (NJ, USA) :Humana Press 2010, 29-58
- CHEW MS, BRIX-CHRISTENSEN V, RAVN HB, et al. Effect of modified ultrafiltration on the inflammatory response in paediatric open-heart surgery: a prospective, randomized study. Perfusion 2002; 17:327-33
- CIRSTOVEANU CG, BARASCU I, McKENZIE STANCU S. Hemadsorption with adult CytoSorb® in a low weight pediatric case. Case Rep Crit Care 2017; 2017:6987 167
- CLARIZIA NA, MANLHIOT C, SCHWARTZ SM, et al. Improved outcomes associated with intraoperative steroid use un high-risk pediatric cardiac surgery. Ann Thorac Surg 2011 ; 91 :1222-7
- DAVIES LK. Cardiopulmonary bypass in infants and children: How it is different ? J Cardiothorac Vasc Anesth 1999; 13:330-45
- DIELMAN JM, NIERICH AP, ROSSEEL PM, et al. Intraoperative high-dose dexamethasone in cardiac surgery: a randomized controlled trial. JAMA 2012 ; 308 :1761-7
- DÖNMEZ A, YURDAKÖK O. Cardiopulmonary bypass in infants. J Cardiothorac Vasc Anesth 2014; 28:778-88
- DU PLESSIS AJ, JONAS RA, WYPIJ D. Perioperative effects of -stat versus pH-stat strategies for deep hypothermic cardiopulmonary bypass in infants. J Thorac Cardiovasc Surg 1997; 114:990-1001
- EATON MP, IANNOLI EM. Coagulation considerations for infants and children undergoing cardiopulmonary bypass. Pediatr Anesth 2011; 21:31-42
- GOLAB HD, SCOHY TV, DE JONG PL, et al. Relevance of colloid oncotic pressure regulation during neonatal and infant cardiopulmonary bypass: a prospective randomized study. Eur J Cardiothorac Surg 2011; 39:886-91
- GRAHAM EM, ATZ AM, McHUGH KE, et al. Preoperative steroid treatment does not improve markers of inflammation after cardiac surgery in neonates: results from a randomized trial. J Thorac Cardiovasc surg 2014; 147:902-8
- GUZZETTA NA, BAJAJ T, FAZLOLLAH T, et al. A comparison of heparin management strategies in infants undergoing cardiopulmonary bypass. Anesth Analg 2008; 106:419-25
- HEYING R, WEHAGE E, SCHUMACHER K, et al. Dexamethasone pretreatment provides antiinflammatory and myocardial protection in neonatal arterial switch operation. Ann Thoarc Surg 2012; 93:869-76
- HIRSCH JC, JACOBS ML, ANDROPOULOS D, et al. Protecting the infant brain during cardiac surgery: a systematic review. Ann Thorac Surg 2012; 94:1365-73
- HOOVER LR, DINAVAHI R, CHENG WP, et al. Jugular venous oxygenation during hypothermic cardiopulmonary bypass in patients at risk for abnormal cerebral autoregulation: influence of -stat versus pH-stat blood gas management. Anesth Analg 2009; 108:1389-93
- JONAS RA. Hypothermia, circulatory arrest, and the pediatric brain. J Cardiothorac Vasc Anesth 1996; 10:66-74
- JONAS RA, WYPIJ D, ROTH SJ, et al. The influence of hemodilution on outcome after 143 JOURNOIS D, POUARD P, GREELEY WJ, et al. Hemofiltration during cardiopulmonary bypass in pediatric cardiac surgery. Anesthesiology 1994; 81:1181-9
- JOURNOIS D, POUARD P, GREELEY WJ, et al. Hemofiltration during cardiopulmonary bypass in pediatric cardiac surgery. Anesthesiology 1994; 81:1181-9
- KERN FH, UNGERLEIDER RM, REVES JG, et al. The effect of altering pump flow rate on cerebral blood flow and cerebral metabolism in neonates, infants and children. Ann Thorac Surg 1993; 56:1366-72
- KURTH CD, STEVEN JM. Keeping a cool head. Anesthesiology 2000; 93:598-600
- MANGOUSH O, PARKAYASTHA S, HAJ-YAHIA S, et al. Heparin-bonded circuits versus nonheparin-bonded circuits. An evaluation of their effect on clinical outcomes. Eur J Cardiothorac Surg 2007 ; 31 :1058-69
- MANLHIOT C, GRUENWALD CE, HOLTBY HM, et al. Challenges with heparin-induced anticoagulation during cardiopulmonary bypass in children: impact of low antithrombin activity. J Thorac Cardiovasc Surg 2016; 151:444-50
- McAULIFFE JJ. Myocardial preservation. In : LAKE CL, ed. Pediatric cardiac anesthesia, 3rd edition. Stamford :Appleton & Lange, 1998, 259-83
- MEDIKONDA R, ONG CS, WADIA R, et al. Trends and updates on cardiopulmlnary bypass setup in pediatric cardiac surgery. J Cardiothorac Vasc Anesth 2019; 33:2804-13
- MUKHERJI J, HOOD RR, EDELSTEIN SB, et al. Overcoming challenges in management of critical events during cardiopulmonary bypass. Semin Cardiothorac Vasc Anesth 2014; 18:190-207
- MURKIN JM, FARAR JK, TWEED WA. Cerebral autoregulation and flow/metabolism coupling during cardiopulmonary bypass: the influence of PaCO2. Anesth Analg 1987; 66:825-32
- NAIK SK, KNIGHT A, ELLIOTT MJ. A prospective randomized study of a modified technique of ultrafiltration during pediatric open-heart surgery. Circulation 1991 ; 84 :III422-31
- PATEL RL, TURTLE MR, CHAMBERS DJ, et al. Alpha-stat acid-base regulation during cardiopulmonary bypass improves neuropsychologic outcomes in patients udergoing coronary artery bypass grapfting. J Thorac Cardiovasc Surg 1996; 111:1267-79
- PEARL JM, LAKS H, DRINKWATER DC, et al. Normocalcemic blood or crystalloid cardioplegia provides better neonatal myocardial protection than low-calcium cardioplegia. J Thorac Cardiovasc Surg 1993 ; 105 :201-6
- POUARD P, MAURIAT P, LABORDE N, BOURDARIAS B. Cicrulation extracorporelle en chirurgie cardiaque pédiatrique chez le nouveau-né, le nourrisson et l’enfant. In: JANVIER G, LEHOT JJ, eds. Circulation extracorporelle: principes et pratique, 2ème édition. Paris: Arnette (Groupe Liaison SA) 2004, 481-506
- RANUCCI M, BALDUINI A, DITTA A, et al. A systematic review of biocompatible cardiopulmonary bypass circuits and clinical outcomes. Ann Thorac Surg 1009 ; 87 :1311-9
- SEIFERT HA, JOBES DR, TEN HAVE T, et al. Adverse events after protamine administratioon following cardiopulmonary bypass in infants and children. Anesth Analg 2003; 97:383-9
- SWAIN JA, McDONALD TJ, ROBBINS RC, et al. Relationship of cerebral and myocardial intracellular pH to blood pH during hypothermia. Am J Physiol 1991 ; 260 :H1640-4
- WANG S, PALANZO D, UNDAR A, et al. Current ultrafiltration techniques before, during and after pediatric cardiopulmonary bypass procedures. Perfusion 2012; 27:438-46
- WARREN OJ, SMITH AJ, ALEXIOU C, et al. The inflammatory response to cardiopulmonary bypass : Part I – Mechanisms of pathogenesis. J Cardiothorac Vasc Anesth 2009 ; 23 :223-31
- WARREN OJ, WATRET AL, DeWIT KL, et al. The inflammatory response to cardiopulmonary bypass : Part II – Anti-inflammatory therapeutic strategies. J Cardiothorac Vasc Anesth 2009 ; 23 :384-93
- WATANABE T, HRITA H, KOBAYASHI M, et al. Brain tissue pH, oxygen tension and carbon dioxide tension in profoundly hypothermic cardiopulmonary bypass. J Thorac Cardiovasc Surg 1989; 97:396-401
- WESLEY MC, PEREIRA LM, SCHARP MA; et al. Pharmakinetics of tranexamic acid in neonates, infants and children undergoing cardiac surgery with cardiopulmonary bypass. Anesthesiology 2015; 122:746-58
- WHITING D, YUKI K, DINARDO JA. Cardiopulmonary bypass in the pediatric population. Best Pract Res Clin Anaesthesiol 2015; 29:241-56
- WHITLOCK RP, CHAN S, DEVEREAUX PJ, et al. Clinical benefit of steroid use in patients undergoing cardiopulmonary bypass : a meta-analysis of randomized trials. Eur Heart J 2008 ; 29 :2592-600
- WHITLOCK RP, DEVEREAUX PJ, TEOH KH, et al. Methylprednisolone in patients undergoing cardiopulmonary bypass (SIRS): a randomised, double-blind, placebo-controlled trial. Lancet 2015; 26:1243-53
- WYPIJ D, JONAS RA, BELLINGER DC, et al. The effect of hematocrit during hypothermic cardiopulmonary bypass in infant heart surgery: results from the combined Boston hematocrit trials. J Thorac Cardiovasc Surg 2008; 135:355-60
- WYPIJ D, NEWBURGER JW, RAPPAPORT LA, et al. The effect of duration of deep hypothermic circulatory arrest in infant heart surgery on late neurodevelopment: the Boston Circulatory Arrest Trial. J Thorac Cardiovasc Surg 2003; 126:1397-403
- YOUNG JN, CHOY IO, SILVA NK, et al. Antegrade cold blood cardioplegia is not demonstrably advantageous over cold crystalloid cardioplegia in surgery for congenital heart disease. J Thorac Cardiovasc Surg 1997 ; 114 :1002-9