In the ventricular segment, a chamber may exhibit variable degrees of hypoplasia, covering a whole range up to complete absence of a ventricle (univentricular heart). Generally, a hypoplastic ventricle presents as an accessory chamber positioned anteriorly if it is a hypoplastic RV or posteriorly if it is a hypoplastic LV (Figure 15.35) (Video).
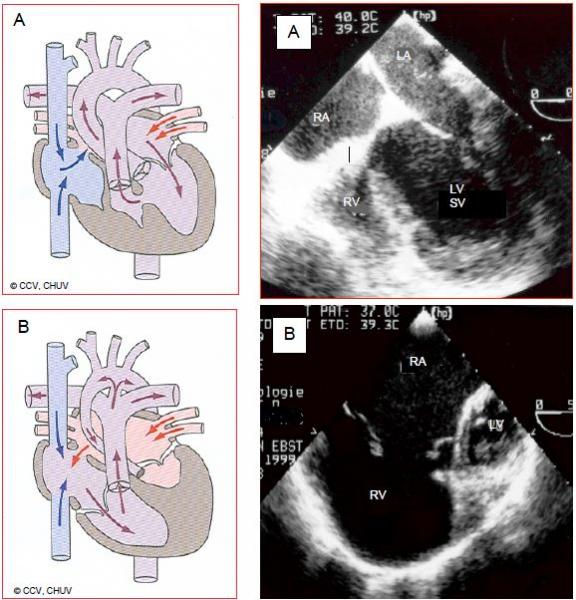
Figure 15.35: Ventricular hypoplasia. A: hypoplastic RV with tricuspid atresia. The RV presents as an accessory anterior chamber connected to the anatomically left single ventricle (SV) via a VSD. Although there is no communication between the RA and RV, there is an ASD between the two atria. B: hypoplastic left heart syndrome. The LV is a small accessory posterior chamber and the ascending aorta is hypotrophic. The SV is anatomically the RV connected to the tricuspid valve and RA. The RA receives the systemic blood via the vena cavae and the pulmonary venous blood via an ASD.
Video: Extreme situation: single ventricle in a child.
The corresponding atrioventricular valve is also hypoplastic, imperforate or absent. The main ventricle (single ventricle) is connected to the aorta and pulmonary artery (double-outlet ventricle). The distribution of flows is dictated by the balance between systemic and pulmonary resistance. Ventricular hypoplasia syndrome comprises various pathologies such as hypoplastic RV with tricuspid atresia, hypoplastic LV and aortic arch, single ventricle of indeterminate morphology, double-inlet LV or RV. The single ventricle (SV) must ensure a double flow for both the systemic and pulmonary circulation. Furthermore, its afterload is high as it is connected to the arterial circuit. Consequently, patients’ outcomes are compromised if the SV is anatomically a right ventricle [3].
The main chamber is subjected to volume and pressure overload. Its degree of remodelling in terms of sphericity has prognostic value for functional development [21]. A shunt at atrial level is crucial for the child’s survival. These patients do not survive into adulthood without surgical intervention, which is usually of a palliative nature [2].
Figure 15.35: Ventricular hypoplasia. A: hypoplastic RV with tricuspid atresia. The RV presents as an accessory anterior chamber connected to the anatomically left single ventricle (SV) via a VSD. Although there is no communication between the RA and RV, there is an ASD between the two atria. B: hypoplastic left heart syndrome. The LV is a small accessory posterior chamber and the ascending aorta is hypotrophic. The SV is anatomically the RV connected to the tricuspid valve and RA. The RA receives the systemic blood via the vena cavae and the pulmonary venous blood via an ASD.
Video: Extreme situation: single ventricle in a child.
The corresponding atrioventricular valve is also hypoplastic, imperforate or absent. The main ventricle (single ventricle) is connected to the aorta and pulmonary artery (double-outlet ventricle). The distribution of flows is dictated by the balance between systemic and pulmonary resistance. Ventricular hypoplasia syndrome comprises various pathologies such as hypoplastic RV with tricuspid atresia, hypoplastic LV and aortic arch, single ventricle of indeterminate morphology, double-inlet LV or RV. The single ventricle (SV) must ensure a double flow for both the systemic and pulmonary circulation. Furthermore, its afterload is high as it is connected to the arterial circuit. Consequently, patients’ outcomes are compromised if the SV is anatomically a right ventricle [3].
The main chamber is subjected to volume and pressure overload. Its degree of remodelling in terms of sphericity has prognostic value for functional development [21]. A shunt at atrial level is crucial for the child’s survival. These patients do not survive into adulthood without surgical intervention, which is usually of a palliative nature [2].
- If the pulmonary circulation is largely open to systemic pressure, PA banding can be used to limit pulmonary blood flow and protect against the effects of PAH;
- If pulmonary blood flow is highly restrictive, a Blalock shunt or ideally a Glenn cavopulmonary anastomosis can be used to improve PA flow. This arrangement is generally followed by a Fontan procedure.
- The rare cases with adequate pulmonary circulation can survive into adulthood.
In adulthood, patients present with a variable degree of cyanosis, numerous arrhythmias, frequent neurological lesions (strokes, brain abscesses) and often have a history of endocarditis. Since the single ventricle must ensure systemic and pulmonary blood flow alone, it dilates and fails after several years, causing atrioventricular valve regurgitation. The reason that this paediatric pathology is mentioned in this chapter is that procedures performed in childhood enable survival into adulthood (82% > 15 years), but impose very unusual haemodynamics (see Chapter 14 Ventricular hypoplasia) [12]. This is notably true of Fontan procedures.
The main challenges for anaesthesia are failure of the single ventricle, which is more common and more difficult to treat if it is an anatomically right ventricle, and maintaining the ratio of PVR to SVR to ensure an optimal balance between pulmonary and systemic blood flow (maintaining stable SaO2). The aim is not to achieve the best possible SaO2, but to ensure an equal aortic and pulmonary flow (Qp/Qs = 1) (see Chapter 14 Ventricular hypoplasia).
Fontan physiology
Several types of Fontan palliation exist. All involve bypassing the tricuspid valve and the RV by diverting central venous blood directly to the pulmonary artery. The single ventricle therefore acts as a systemic ventricle, resulting in two circulations in series (Figure 15.36) [14].
The main challenges for anaesthesia are failure of the single ventricle, which is more common and more difficult to treat if it is an anatomically right ventricle, and maintaining the ratio of PVR to SVR to ensure an optimal balance between pulmonary and systemic blood flow (maintaining stable SaO2). The aim is not to achieve the best possible SaO2, but to ensure an equal aortic and pulmonary flow (Qp/Qs = 1) (see Chapter 14 Ventricular hypoplasia).
Fontan physiology
Several types of Fontan palliation exist. All involve bypassing the tricuspid valve and the RV by diverting central venous blood directly to the pulmonary artery. The single ventricle therefore acts as a systemic ventricle, resulting in two circulations in series (Figure 15.36) [14].
- A single ventricle pumping oxygenated blood into the systemic circulation;
- A central venous system supplying the pulmonary circulation without assistance from a pump (non-pulsatile flow).
Figure 15.36: Tricuspid atresia and its palliation by Fontan. A: The tricuspid valve is a fibromuscular membrane (green arrow), and the RV is hypoplastic and communicates with the LV via a small VSD (blue arrow). The LV is the functional single ventricle (SV). It receives blood from the LA via the mitral valve and communicates with the aorta and PA. B: The venous blood flows from the RA to the LA via an ASD and mixes with arterialised blood from the pulmonary veins. This mixture is expelled into the aorta by the single ventricle. C: Fontan lateral tunnel – blood from the inferior vena cava (IVC) drains into the RA and subsequently into the pulmonary artery (PA) via the anastomosis between the SVC root and the right pulmonary artery (RPA). The ASD acts as a relief valve in the event of high pressure. D: external Fontan conduit (prosthetic tube) routing blood from the IVC to the PA; It is often fenestrated in the RA to avoid overload. In C and D, the Glenn shunt (GS) is a end-to-side anastomosis of the superior vena cava (SVC) to the right pulmonary artery (RPA).
The first stage is the Glenn procedure, which entails anastomosing the superior vena cava (SVC) to the right pulmonary artery (RPA), which is separated from the PA trunk (unidirectional shunting) or anastomosing it laterally near the pulmonary bifurcation, maintaining PA continuity (bidirectional shunting). In children, the SVC drains half of the systemic venous return, giving a Qp/Qs ratio of 0.5, while in adults, it drains less than a third of this (Qp/Qs 0.3). The second stage (1-3 years later) is the Fontan procedure, which has undergone several changes since it was first described in 1971: direct anastomosis of the RA to the PA (abandoned), lateral tunnel connecting the IVC to the PA via the SVC or prosthetic external conduit from the IVC to the PA [7,15,16,17]. The RA-PA anastomosis results in significant dilation of the RA, which increases the risk of arrhythmia and thrombosis. It is no longer practised. The lateral tunnel created by the IVC → RA → SVC → PA conduit offers the benefit of growing with the child. However, the external IVC → PA conduit provides better haemodynamics and maintains lower LAP. By fenestrating the Fontan conduit in the atrium (Figure 15.36D), it is possible to limit the risk of excessive pressure in the central venous circuit and maintain preload in the systemic ventricle in the event of an increase in PVR and a reduction in pulmonary blood flow. However, this comes at the cost of some arterial desaturation [6]. The surgical mortality rate of Fontan in paediatrics is currently < 5%, but that of reoperation in adulthood is 11-15% [2,18]. Survival is > 90% at 10 years and ≥ 80% at 20 years [2,8,10,12,18,23]. Better outcomes are observed in cases of hypoplastic RV, since the single systemic ventricle in such instances is an anatomically left ventricle.
The haemodynamics of the Fontan circulation are characterised by an entirely passive pulmonary flow, whose flow rate is solely dependent on central venous pressure. The CVP must therefore be kept high (≥ 15 mmHg). Certain conditions are required for a Fontan procedure to work.
- An adequate pulmonary vascular bed;
- Low pulmonary vascular resistance (PVR);
- Low LAP (5-8 mmHg);
- A competent atrioventricular valve;
- A sinus rhythm;
- A systemic ventricle with good systolic and diastolic function.
Left atrial pressure must not exceed 5-8 mmHg, as a transpulmonary gradient of 6-10 mmHg is necessary to ensure the flow between the RA and LA [5]. Any increase in PVR (hypoxia, atelectasis, pneumonia), intrathoracic pressure (Valsalva, IPPV) or LAP (left-sided failure, atrioventricular insufficiency, non-sinus rhythm) dramatically reduces pulmonary blood flow, thereby also reducing systemic ventricle preload. Arterial oxygen saturation is normally 90-95% at room air. If saturation falls below 90%, this indicates insufficient pulmonary blood flow. Exercise tolerance is half of that of adults of the same age [11].
Fontan physiology is unusual since the interposition of a functional pump between the systemic venous return and the pulmonary circulation is lost. The single ventricle is affected by excessive afterload rather than volume overload (cardiac output is normal) as it must provide sufficient kinetic energy for a longer course than the systemic circuit. This circuit includes both systemic resistance (SVR) and pulmonary resistance (PVR) (see Figure 14.54). It must also overcome resistance created by the cavopulmonary anastomosis and loss of energy due to the collision of flows from the SVR, IVC and right PA. Afterload is further increased by the need to maintain high central venous pressure. Consequently, the LV develops more energy (+75%) to produce the same flow as in a normal circuit. LV preload is limited since the venous capacitance is reduced to maintain sufficient venous pressure. Combined with the loss of compliance of the pulmonary vascular bed, which is already maximally vasodilated, this limitation of preload is the reason why it is impossible to increase cardiac output on exercise in patients with a Fontan circuit [14]. The loss of pulsatility in the pulmonary vascular bed reduces NO production as this is linked to cyclic wall stress on the pulmonary vascular wall. Unfortunately, this phenomenon contributes to an increase in PVR [16].
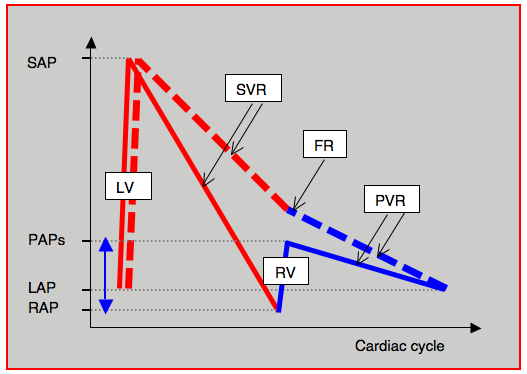
Figure 14.54: Diagram of hydraulic energy supplied by the single ventricle to the Fontan circulation and represented here by intravascular pressure. Normally (solid curves), the LV causes pressure to rise from LA pressure (LAP) to peak systemic arterial pressure (SAP). In line with systemic vascular resistance (SVR), the pressure drops back down to the level of right atrial pressure (RAP). The RV then raises it again to the level of systolic pulmonary arterial pressure (PAPs) after which it falls again, in line with pulmonary vascular resistance (PVR), to the value of left atrial pressure. In the Fontan circulation (dashed curve), the energy supplied by the RV (blue double arrow) is no longer present. It is dependent on the LV, which must pump the blood beyond the systemic venous circuit into the pulmonary circulation. FR: resistance in the Fontan conduit. Red line: left circulation. Blue line: right circulation.
The flow velocity in a Fontan conduit is approximately 0.2 – 0.5 m/s, which is the same as in the great central veins. In the absence of a ventricular pump, the blood flow in it is pulseless. It oscillates during the cardiac cycle with a systolic component and a diastolic component (Figure 15.37).
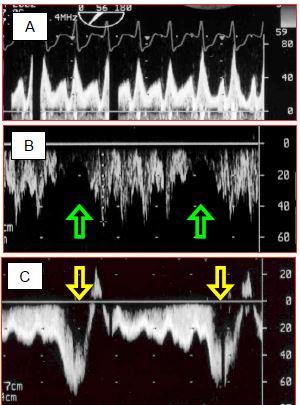
Figure 15.37: Spectral Doppler recordings of flow in a Fontan circuit. A: pulmonary flow in a Fontan circulation – the flow is of a central venous rather than arterial type, with two clearly separated systolic and diastolic components. Vmax is normal for a central vein (< 0.8 m/s). B: with IPPV, the flow is interrupted during each positive-pressure inspiration of the ventilator. C: in contrast during spontaneous respiration, the flow accelerates considerably during inspiration, when intrathoracic pressure falls below atmospheric pressure. This provides valuable assistance for the pulmonary blood flow. In B and C, flow is recorded through the transgastric route, which explains why the flow is below the baseline (it moves away from the transducer when flowing from the IVC to the PA).
High velocity (> 1.5 m/s) indicates stenosis, while a loss of oscillation suggests obstruction [9]. Mean pulmonary blood flow is largely dependent on the increase in flow occurring at each inspiration during spontaneous respiration. In contrast, during IPPV, the flow is reduced or even interrupted with each ventilator inspiration.
Although successful in some respects, the Fontan system does not guarantee long-term normal survival. Failure is manifested by dyspnoea, cyanosis, fluid retention, palpitations and syncopes. Multiple causes can be identified [6,20].
Fontan physiology is unusual since the interposition of a functional pump between the systemic venous return and the pulmonary circulation is lost. The single ventricle is affected by excessive afterload rather than volume overload (cardiac output is normal) as it must provide sufficient kinetic energy for a longer course than the systemic circuit. This circuit includes both systemic resistance (SVR) and pulmonary resistance (PVR) (see Figure 14.54). It must also overcome resistance created by the cavopulmonary anastomosis and loss of energy due to the collision of flows from the SVR, IVC and right PA. Afterload is further increased by the need to maintain high central venous pressure. Consequently, the LV develops more energy (+75%) to produce the same flow as in a normal circuit. LV preload is limited since the venous capacitance is reduced to maintain sufficient venous pressure. Combined with the loss of compliance of the pulmonary vascular bed, which is already maximally vasodilated, this limitation of preload is the reason why it is impossible to increase cardiac output on exercise in patients with a Fontan circuit [14]. The loss of pulsatility in the pulmonary vascular bed reduces NO production as this is linked to cyclic wall stress on the pulmonary vascular wall. Unfortunately, this phenomenon contributes to an increase in PVR [16].
Figure 14.54: Diagram of hydraulic energy supplied by the single ventricle to the Fontan circulation and represented here by intravascular pressure. Normally (solid curves), the LV causes pressure to rise from LA pressure (LAP) to peak systemic arterial pressure (SAP). In line with systemic vascular resistance (SVR), the pressure drops back down to the level of right atrial pressure (RAP). The RV then raises it again to the level of systolic pulmonary arterial pressure (PAPs) after which it falls again, in line with pulmonary vascular resistance (PVR), to the value of left atrial pressure. In the Fontan circulation (dashed curve), the energy supplied by the RV (blue double arrow) is no longer present. It is dependent on the LV, which must pump the blood beyond the systemic venous circuit into the pulmonary circulation. FR: resistance in the Fontan conduit. Red line: left circulation. Blue line: right circulation.
The flow velocity in a Fontan conduit is approximately 0.2 – 0.5 m/s, which is the same as in the great central veins. In the absence of a ventricular pump, the blood flow in it is pulseless. It oscillates during the cardiac cycle with a systolic component and a diastolic component (Figure 15.37).
Figure 15.37: Spectral Doppler recordings of flow in a Fontan circuit. A: pulmonary flow in a Fontan circulation – the flow is of a central venous rather than arterial type, with two clearly separated systolic and diastolic components. Vmax is normal for a central vein (< 0.8 m/s). B: with IPPV, the flow is interrupted during each positive-pressure inspiration of the ventilator. C: in contrast during spontaneous respiration, the flow accelerates considerably during inspiration, when intrathoracic pressure falls below atmospheric pressure. This provides valuable assistance for the pulmonary blood flow. In B and C, flow is recorded through the transgastric route, which explains why the flow is below the baseline (it moves away from the transducer when flowing from the IVC to the PA).
High velocity (> 1.5 m/s) indicates stenosis, while a loss of oscillation suggests obstruction [9]. Mean pulmonary blood flow is largely dependent on the increase in flow occurring at each inspiration during spontaneous respiration. In contrast, during IPPV, the flow is reduced or even interrupted with each ventilator inspiration.
Although successful in some respects, the Fontan system does not guarantee long-term normal survival. Failure is manifested by dyspnoea, cyanosis, fluid retention, palpitations and syncopes. Multiple causes can be identified [6,20].
- Atrial tachyarrhythmias are common (40-50% at 20 years) and poorly tolerated as they increase the pulmonary circuit’s downstream pressure [6,22]. They are often associated with atrial dilation.
- The single ventricle must ensure the systemic circulation and supply the energy required to maintain sufficient central venous pressure for the pulmonary circulation. If its function deteriorates, its diastolic pressure increases, which raises the downstream pressure of the pulmonary vascular bed and immediately slows pulmonary blood flow. Ventricular failure is evident in 50% of patients above the age of 30 years [19]. Since the preload reserve is low, blood flow mainly increases through tachycardia.
- Since CVP must remain high to ensure pulmonary blood flow, systemic venous stasis is common. Twenty-eight percent of patients present with fluid retention, hepatomegaly, ascites, and protein-losing enteropathy [8]. These side effects, which may be limited by fenestrating the Fontan conduit, are associated with protein loss and liver dysfunction, which may result in long-term cirrhosis. This culminates in hypoproteinaemia and significant changes in clotting factor synthesis entailing a significant risk of haemorrhage [24].
- Due to venous hypertension and low systemic blood flow, the incidence of renal dysfunction is 50% in adult Fontan patients [4].
- The mean incidence of thromboembolism is 25% and that of stroke is 3-20% [6,25].
- Since normal saturation at room air is only 90-95%, the slightest fall in pulmonary blood flow causes cyanosis.
In order to ensure optimal haemodynamics through the pulmonary vascular bed, patients are generally administered phosphodiesterase-5 inhibitors (sildenafil, tadalafil), endothelin receptor antagonists (bosentan, ambrisentan), anti-inflammatory agents (budesonide), antiplatelet agents (aspirin), and anticoagulants in the event of thromboembolism. All these drugs must be administered perioperatively without interruption in the event of surgery.
Anaesthesia in the event of Fontan
Anaesthetists should encourage pulmonary flow, which is passive, and maintain systemic ventricle function [6,13,16]. Low cardiac output is largely due to reduced preload (hypovolaemia), raised PVR, ventricular dysfunction, or arrhythmias.
Anaesthesia in the event of Fontan
Anaesthetists should encourage pulmonary flow, which is passive, and maintain systemic ventricle function [6,13,16]. Low cardiac output is largely due to reduced preload (hypovolaemia), raised PVR, ventricular dysfunction, or arrhythmias.
- PVR must remain low in order to maintain blood flow through the lungs. Acidosis, hypoxaemia and hypercapnia must therefore be avoided. It is essential to maintain hypocapnia (PaCO2 35 mmHg, pH 7.45) through moderate hyperventilation. FiO2 is adjusted to 0.5-0.8.
- PVR may be reduced by hypobaric hyperventilation, inhalation of NO (10-20 ppm), prostaglandins, a PDE5 inhibitor such as sildenafil, or an inodilator such as milrinone.
- Inspiration during spontaneous respiration draws the blood into the lungs, which increases the pulmonary blood flow. In contrast, controlled ventilation reduces or interrupts the pulmonary blood flow at each ventilator inspiration (Figure 15.37C). Therefore, where possible, these patients should be kept in spontaneous ventilation, provided that they are not hypoventilating. If IPPV is required, the mean ventilation pressure should be kept as low as possible. The best approach to ventilation is to prevent hypoxia, hypercapnia and atelectasis, and keep mean intrathoracic pressure at minimal levels (hypobaric hyperventilation). The adequacy of blood flow in the Fontan circuit may be monitored by TEE.
- Hypovolaemia is extremely poorly tolerated since the reduction in preload instantly reduces the pulmonary blood flow (fall in CVP). Tachycardia is the sole means of increasing cardiac output. However, anaesthesia reduces LV preload due to the limitation of pulmonary blood flow by IPPV and venodilation typical of reduced sympathetic tone (drop in CVP).
- Ventricular function is generally limited and usually requires inotropic stimulation. Milrinone and epinephrin is the most effective combination. Any deterioration in systemic ventricular function increases the pressure in the LA and reduces the transpulmonary gradient, thereby also reducing pulmonary blood flow. Anaesthetic agents should be chosen on the basis of this poor ventricular performance.
- The central venous line (CVP) actually measures pulmonary arterial driving pressure (PAP). It is very useful to monitor this measurement, but the risk of thrombosis is high. The catheter must be removed as soon as possible. It is less dangerous to measure venous pressure in the external jugular vein (short 14-16G Venflon-type catheter) or in the femoral vein (20 cm 14-16G catheter). TEE offers a less invasive and safer means of monitoring preload. CVP must be kept at approximately 15 mmHg.
- Recommended monitoring: SpO2, ECG, arterial catheter, venous pressure, ETCO2, ScO2, TEE if possible.
- During induction, propofol and midazolam dangerously lower preload – etomidate is a safer agent. Thiopental is a cardiodepressant and ketamine increases PVR in adults – these agents are contraindicated.
- Neuraxial blockade, especially epidural, is a good solution provided that care is taken to avoid any hypovolaemia, which could lower pulmonary perfusion pressure, by ensuring very gradual installation of sympathetic blockade.
- In the postoperative phase, spontaneous breathing should be resumed as quickly as possible as well as anticoagulation or prophylactic aggregation inhibition. Any nausea and vomiting should be avoided due to the increase in intrathoracic pressure during Valsalva manoeuvres.
An elective non-cardiac operation is only considered if the patient is well balanced. Bariatric surgery is difficult or even dangerous in patients with a Fontan circuit, since high abdominal pressure impedes venous return, CO2 absorption leads to hypercapnia and therefore increased PVR, and the risk of paradoxical gas embolism is high in the event of fenestration [16]. It is possible depending on the patient's tolerability and provided abdominal pressure is kept < 10 mmHg.
© BETTEX D, CHASSOT PG, January 2008, last update February 2020
Fontan circulation |
Characteristics:
- Bypassing of the RV by diverting the SVC + IVC → PA - Reduced pulmonary flow (Qp ↓); driving pressure: CVP - Pulmonary perfusion pressure: CVP - LAP (ΔP min: 6-10 mmHg) - Normal SpO2: 90-95% - Flow dependent on preload Functional conditions: - CVP ≥ 15 mmHg - LAP < 8 mmHg - Low PVR - Low intrathoracic P - Normal LV and atrioventricular valve function - No arrhythmia Sequelae: arrhythmias, venous stasis, ascites, cirrhosis, thrombosis, LV dysfunction Anaesthesia recommendations: - Spontaneous ventilation if possible – avoid any hypoventilation - Ventilation: FiO2 ↑, hypocapnia, alkalosis, low intrathoracic mean P, no PEEP, NO - Neuraxial blockade suitable - Hypovolaemia poorly tolerated (reduction in pulmonary blood flow due to lower CVP) |
© BETTEX D, CHASSOT PG, January 2008, last update February 2020
References
- BALAJI S, GEWILLIG M, BULL C, et al. Arrhythmias after Fontan procedure: comparison of total cavopulmonary connection to atriopulmonary connection. Circulation 1991; 84(Suppl III):162-7
- BAUMGARTNER H, BONHOEFFER P, DE GROOT NMS, et al. ESC Guidelines for the management of grown-up congenital heart disease (new version 2010). Eur Heart J 2010; 31:2915-57
- BHATT AB, FOSTER E, KUEHL K, et al. Congenital hesart disease in older adult. A Scientific Statement from the American Heart Association. Circulation 2015; 131:1884-931
- DIMOPOULOS K, DILLER GP, KOLTSIDA E, et al. Prevalence, predictors and prognostic value of renal dysfunction in adults with congenital heart disease. Circulation 2008; 117:2320-8
- DISSESSA TG, CHILD JS, PERLOFF JK, et al. Systemic venous and pulmonary arterial flow patterns after Fontan’s procedure for tricuspid atresia or single ventricle. Circulation 1984; 70:898-902
- EAGLE SS, DAVES SM. The adult with Fontan physiology: systematic approach to perioperative management for noncardiac surgery. J Cardiothorac Vasc Anesth 2011; 25:320-34
- FONTAN F, BAUDET E. Surgical repair of tricuspide atresia. Thorax 1971; 26:240
- FONTAN F, KIRKLIN JW, FERNANDEZ G, et al. Outcome after a "perfect" Fontan operation. Circulation 1990; 81:1520-36
- FYFE DA, KLINE CH, SADE RM, GREENE CA, GILETTE PC. The utility of transesophageal echocardiography during and after Fontan operations in small children. Am Heart J 1991; 122:1403-15
- GENTLES TL, MAYER JE Jr, GAUVREAU K, et al. Fontan operation in five hundred consecutive patients: factors influencing early and late outcome. J Thorac Cardiovasc Surg 1997; 114:376-91
- HARRISON DA, LIU P, WALTERS JE, et al. Cardiopulmonary function in adult patients late after Fontan repair. J Am Coll Cardiol 1995; 26:1016-21
- HOSEIN RB, CLARK AJ, McGUIRK SP, et al. Factors influencing early and late outcome following the Fontan procedure in the current era. Eur J Cardiothorac Surg 2007; 31:344-53
- HOSKING MP, BEYNEN FM. The modified Fontan procedure: physiologic and anesthetic implications. J Cardiothorac Vasc Anesth 1992; 6:465-7
- JOLLEY M, COLAN SD, RHODES J, DINARDO J. Fontan physiology revisited. Anesth Analg 2015; 121:172-82
- KUMAR SP, RUBENSTEIN CS, SIMSIC JM, et al. Lateral tunnel versus extracardiac conduit Fontan procedure: a concurrent comparison. Ann Thorac Surg 2003; 76:1389-97
- LEYVI G, WASNICK JD. Single-ventricle patient: pathophysiology and anesthetic management. J Cardiothorac Vasc Anesth 2010; 24:121-30
- MARCELETTI CF, HANLEY FL, MAVROUDIS C, et al. Revisions of previous Fontan connections to total extracardiac cavopulmonary anastomosis: A multicenter experience. J Thorac Cardiovasc Surg 2000; 119:340-6
- MASCIO CE, PASQUALI SK, JACOBS JP, et al. Outcomes in adult congenital heart surgery: analysis of the Society of Thoracic Surgeons database. J Thorac Cardiovasc Surg 2011; 142:1090-7
- NOROSI K, WESSEL A, ALPERS V, et al. Incidence and risk distribution of heart failure in adolescents and adults with congenital heart disease after cardiac surgery. Am J Cradiol 2006; 97:1238-43
- RYCHIK J, GOLDBERG DJ. Late consequences of the Fontan operation. Circulation 2014; 130:1525-8
- SANO T, OGAWA M, TANIGUCHI K, et al. Assessment of ventricular contractile state and function in patients with univentricular heart. Circulation 1989; 79:1247-56
- STAMM C, FRIEHS I, MAYER JE, et al. Long-term results of the lateral tunnel Fontan operation. J Thorac Cardiovasc Surg 2000; 121:28-41
- D’UDEKEM Y, IYENGAR AJ, COCHRANE AD, et al. The Fontan procedure: contemporary techniques have improved long-term outcomes. Circulation 2007; 116:157-64
- VAN NIEUWENHUIZEN RC, PETERS M, LUBBERS TJ, ET AL. Abnormalities in liver function and coagulation profile following the Fontan procedure. Heart11999; 82:40-6
- VARMA C, WARR MR, HENDLER AL, et al. Prevalence of "silent" pulmonary emboli in adults after the Fontan operation. J Am Coll Cardiol 2003; 41:2252-8