Several pathologies contribute to a situation in which circulation is provided by a single ventricle (univentricular heart). In such cases, the other ventricle is reduced to an accessory chamber positioned anteriorly in case of hypoplastic RV or posteriorly in case of hypoplastic LV. Functionally, this situation is equivalent to that of a single ventricle (SV) (Figure 14.46). The AV valve of the hypoplastic ventricle is often atretic, but the single functional ventricle may receive blood from two AV valves (double inlet ventricle). The SV ejects the stroke volume of mixed blood directly into the aorta and PA (double outlet ventricle) or via a VSD or patent ductus arteriosus. Survival is not possible in this specific physiology for three reasons: the patient is cyanotic due to the mix of circulations; the ventricle is subjected to volume overload, as it must by itself provide both the systemic and pulmonary blood flow; and it is impossible to continuously maintain an adequate Qp/Qs ratio [11].
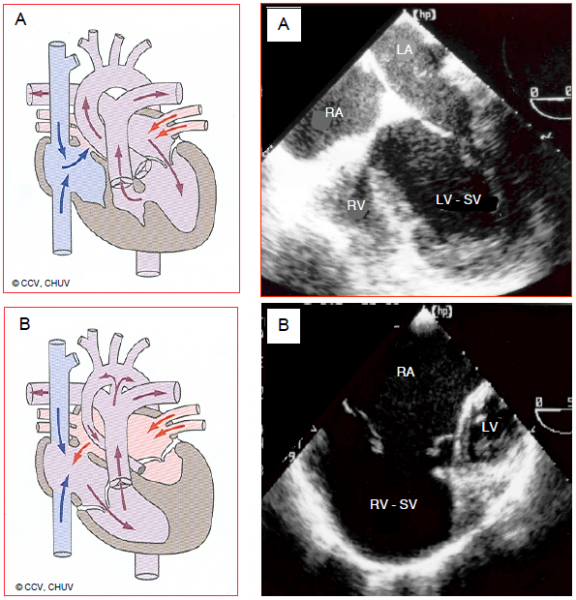
Figure 154.46: Ventricular hypoplasia. A: hypoplastic RV with tricuspid atresia. The RV presents as an accessory anterior chamber connected to the anatomically left single ventricle (SV) via a VSD. Although there is no communication between the RA and RV, there is an ASD between the two atria. B: hypoplastic left heart syndrome. The LV is a small accessory posterior chamber and the ascending aorta is hypotrophic. The SV is the anatomical RV connected to the tricuspid valve and RA. The RA receives the systemic blood via the vena cavae and the pulmonary venous blood via an ASD.
Single ventricle syndrome may be divided into three elements.
Figure 154.46: Ventricular hypoplasia. A: hypoplastic RV with tricuspid atresia. The RV presents as an accessory anterior chamber connected to the anatomically left single ventricle (SV) via a VSD. Although there is no communication between the RA and RV, there is an ASD between the two atria. B: hypoplastic left heart syndrome. The LV is a small accessory posterior chamber and the ascending aorta is hypotrophic. The SV is the anatomical RV connected to the tricuspid valve and RA. The RA receives the systemic blood via the vena cavae and the pulmonary venous blood via an ASD.
Single ventricle syndrome may be divided into three elements.
- Tricuspid atresia and hypoplastic RV; the pulmonary flow is dependent on the extent to which the valve and pulmonary artery are hypoplastic and the presence of a patent ductus arteriosus.
- Hypoplastic left heart syndrome; whatever the degree of mitral and aortic valve involution, the LV and ascending aorta are always hypoplastic. The systemic flow is dependent on the patent ductus arteriosus, which discharges blood from the PA into the aorta.
- Coexistence of several lesions (very wide VSD, extensive straddling) rendering septation impossible, even if two separate and functional ventricles are present.
The aim of surgery is to restore the Qp/Qs ratio to approximately 1:1, ensure satisfactory systemic flow, and create conditions enabling PVR to be lowered. Two different types of surgery are initially possible, depending on whether pulmonary flow is insufficient or excessive.
- In the event of a hypoplastic LV: a systemic-pulmonary shunt (Blalock-Taussig shunt or Sano shunt, which is a SV-PA tube) is established to increase pulmonary flow using a tubular prosthetic graft. The Qp/Qs ratio is dependent on the size of this shunt and on arterial and pulmonary resistances. At the same time, the hypoplastic aorta is reconstructed to increase systemic flow (Stage 1 Norwood = Damus-Kaye-Stansel procedure).
- In the event of a hypoplastic RV: the pulmonary artery is narrowed with a Teflon™ band (banding or FloWatch®) to reduce pulmonary flow if this is not restrictive. Banding tightness is adjusted based on SpO2.
Tricuspid atresia and hypoplastic RV
In this third most common neonatal cyanotic heart disease after tetralogy of Fallot (TOF) and transposition of the great arteries (TGA), the RA does not communicate with the RV, which is hypoplastic since the tricuspid valve is imperforate. The central venous blood flows into the LA via an ASD. The left heart is overloaded since it must pump the stroke volume for both the systemic and the pulmonary circulation (Figure 14.47). In a third of all cases, the great arteries are transposed.
Figure 14.47: Tricuspid atresia and hypoplastic RV. A: The tricuspid valve is a fibromuscular membrane (green arrow), and the RV is hypoplastic and communicates with the LV via a small VSD (blue arrow). The LV is the functional single ventricle (SV). It receives blood from the LA via the mitral valve and communicates with the aorta and PA. B: The venous blood flows from the RA to the LA via an ASD and mixes with arterialised blood from the pulmonary veins. This mixture is expelled into the aorta and PA by the single ventricle.
Hypoplastic left heart syndrome
This term covers a set of pathologies that are more or less the opposite of the previously described anatomy: the LV is small and non-functional and the mitral valve, aortic valve, ascending aorta and aortic arch are affected by hypoplasia to varying degrees or by complete atresia. The aortic arch may even be interrupted. The systemic and pulmonary venous returns mix in the RA. The RV supplies the pulmonary and systemic circulation via the patent ductus arteriosus (Figure 14.46B). As soon as the patent ductus arteriosus flow decreases, the systemic flow plummets and the infant dies within 3-5 days.
Physiological management of univentricular heart defects
The pulmonary to systemic flow ratio (Qp:Qs) is the key factor in managing children with univentricular heart defects. The aim is to achieve flows that are as close as possible to equilibrium (Qp:Qs = 1), in order to maintain satisfactory systemic O2 delivery (DO2). Since PVR falls below SVR within days of birth, the pulmonary circuit tends to divert blood from the systemic circuit, especially if patients are hyperventilated in order to optimise SaO2. This results in systemic hypoperfusion, arterial hypotension, a reduction of coronary blood flow and metabolic acidosis. Therapy involves preventing excessive pulmonary blood flow by increasing PVR and hypoventilating the patient, rather than administering a vasopressor. SaO2 of 75-80% indicates balanced Qp:Qs, provided that pulmonary venous saturation (SvpO2) is > 95% and central mixed venous saturation (SvO2) is > 50% [26]. The former is measured by a catheter placed surgically in the LA and the latter by a central line with its tip at the SVC-RA junction.
The SaO2 / (SaO2 - SvO2) ratio or Ω ratio (oxygen excess factor), reflects the excess of delivered O2 in relation to O2 actually consumed. It is the opposite of the O2 extraction ratio. It enables optimal adjustment of the Qp/Qs ratio in these extremely frail infants and is the best means of monitoring DO2 [3,32]. This Ω ratio has a linear relationship with DO2 for a given O2 requirement. Any surgery that raises it is beneficial.
The current assumption is that O2 delivery (DO2) is more dependent on SVR and haemoglobin levels than on PVR and pulmonary blood flow. Moreover, it is easier to adjust SVR than PVR as PVR is always high in neonates. Therefore, the focus tends to be mainly on lowering SVR and VO2 when seeking to optimise the Qp:Qs ratio [18,24]. DO2 is optimal if Qp/Qs is < 1, but falls if Qp/Qs is > 1. DO2 correlates more with SvO2 than with SaO2. Anaerobic metabolism and systemic acidosis are generally triggered if SvO is < 35% [26]. Intensive care is entirely focused on vascular resistance, ventricular function and Hb levels in order to ensure an optimal systemic O2 delivery. Systemic haemodynamics are controlled preoperatively by a vasodilator infusion (phenoxybenzamine, sodium nitroprusside) and administration of a beta-blocker (bisoprolol po) or ACE inhibitor (lisinopril po) [36]. In the operating theatre, the child is ventilated with low FiO2 (0.21-0.3) and hypercapnia (PaCO2 45-50 mmHg) for SaO2 of 75-80%. Greater oxygenation (SaO2 > 80%) would equate to excessive pulmonary flow and result in a state of systemic shock. Inotropic support (dobutamine, epinephrin, milrinone) is required [27].
The functional ventricle is subjected to volume overload since it is required for both the systemic and pulmonary circulation. It dilates and increases its wall stress. Moreover, diastolic pressure is low due to constant leakage of volume into the pulmonary circulation. These two phenomena combine to increase the risk of subendocardial ischaemia faced by infants as soon as they become tachycardic or hypervolaemic. At this age, acute ischaemia leads to ventricular fibrillation. Furthermore, in cases of aortic hypoplasia, the coronary arteries are retrogradely perfused by blood from the patent ductus arteriosus that must flow through an ascending aorta sometimes measuring as little as 1-2 mm in diameter.
Initial surgical strategies
Since neonates depend on the patent ductus arteriosus (PDA) for pulmonary perfusion (tricuspid atresia, hypoplastic right heart) or for systemic perfusion (interrupted aortic arch, hypoplastic left heart), the PDA must be kept open by continuous administration of prostaglandin E1 (0.01-0.05 mcg/kg/min) from birth until semi-urgent surgery is performed. If the atrial septum is intact or if the ASD is too restrictive, a Rashkind septostomy is performed at birth.
The first surgical step is initial palliation aimed at ensuring the infant’s survival for several months. Depending on whether the pulmonary flow is insufficient or excessive, the following is techniques are initially performed [26]:
- Narrowing of the pulmonary artery with a Teflon™ band (banding) to reduce the pulmonary flow if it is higher than the systemic flow. Banding tightness is adjusted based on the SpO2 level achieved. PAP is reduced to a third of systemic AP.
- Pulmonary banding is contraindicated if the systemic flow is dependent on flow from the PA via the patent ductus arteriosus as is the case in aortic hypoplasia (see Figure 14.46B). In this case, a Damus-Kaye-Stansel procedure is performed (see Figure 14.68).
- A systemic-pulmonary shunt (modified Blalock-Taussig shunt - MBTS) aimed at increasing pulmonary flow. Depending on the position of the aortic arch, the subclavian artery is connected to the right or left pulmonary artery with a prosthetic tubular graft of 3-4 mm in diameter. The Qp/Qs ratio is dependent on the size of this shunt and on arterial and pulmonary resistances (Figure 14.48).
Figure 14.48: Blalock-Taussig shunt. A: standard shunt – the left subclavian artery is sacrificed and anastomosed end-to-side to the left branch of the pulmonary artery. B: modified shunt – the connection is established with a GoreTex™ tube (3-4 mm diameter) between the subclavian artery and the right or left PA. In this case, the subclavian flow for the arm is kept intact. LCA: left carotid artery LSCA: left subclavian artery. BCA: brachiocephalic artery. MBTS: Modified Blalock-Taussig shunt.
If systemic flow is insufficient (hypoplastic left heart and aorta), multiple-step reconstruction techniques are possible, with the first step performed in the first days or weeks of life.
- The Stage 1 Norwood procedure consists of: atrial septostomy, widening of the aortic arch, anastomosis between the proximal PA and the aorta, closure of the distal PA and creation of a distal Blalock-Taussig shunt (Figure 14.49A). The systemic circulation is now dependent on the single ventricle and not the PDA, which is ligated. By interrupting the PA trunk, pulmonary flow is made dependent on a distal shunt whose small diameter (3.0 mm) ensures low PAP. Previously, the operation was generally performed under CPB with deep hypothermia and circulatory arrest, which is rarely the case nowadays.
- The Sano procedure involves the same widening of the aortic arch, but uses a shunt between the RV and PA (5-6 mm diameter) instead of a Blalock shunt to ensure pulmonary flow (Figure 14.49B). Its benefits are higher diastolic pressure, a lack of competition with the coronary flow, and pulsatile pulmonary flow. The procedure entails more immediate complications, but superior results at 12 months (74% without complications) [30].
- Hybrid procedures such as the Giessen technique are extracardiac operations including percutaneous catheterisation and surgery without CPB (Figure 14.50) [1,36].
- Bilateral narrowing (banding) of the PA by sternotomy,
- Dilation and stenting of the patent ductus arteriosus by catheterisation,
- Atrial septostomy (Rashkind) by catheterisation.
This procedure is simpler than the stage 1 Norwood procedure – the mortality rate is < 1% [36].
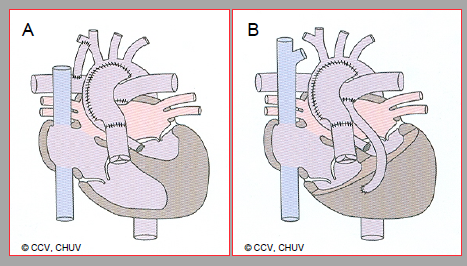
Figure 14.49: First surgical step in the event of hypoplastic left heart. A: palliation according to Norwood (stage 1). This entails: widening of the aortic arch, anastomosis between the proximal PA and aorta, closure of the distal PA and creation of a distal Blalock-Taussig shunt (right subclavianRPA). B: Modified Norwood according to Sano. A conduit between the RV and PA replaces the Blalock-Taussig shunt.
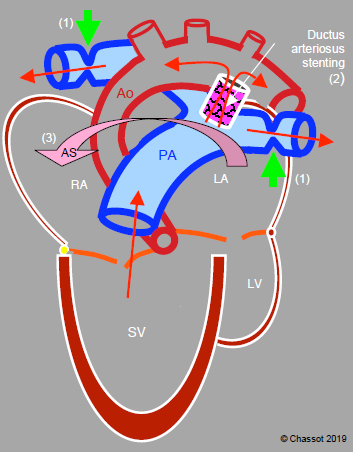
Figure 14.50: Hybrid technique (Giessen procedure) in the event of a hypoplastic left heart and ascending aorta: 1) banding of the right and left pulmonary arteries (green arrows), 2) ductus arteriosus patency maintained by a stent, 3) atrial septostomy (AS arrow) to facilitate mixture of the two circulations. Stenting of the ductus arteriosus and septostomy are achieved by percutaneous catheterisation, while the two bandings are achieved surgically by median sternotomy. These procedures are extracardiac and extrapericardial. Therefore, the single ventricle provides the pulmonary and systemic flow via the ductus arteriosus, while the pulmonary circulation is protected from high pressure by the two bandings.
"Permanent” palliative surgery
If it is not possible to reconstruct two functional chambers due to ventricular hypoplasia, iterative palliative procedures are necessary (univentricular repair). The single functional ventricle is used to ensure high-pressure systemic circulation. The challenge is then to create a set up to ensure pulmonary circulation at a 5 times lower pressure regime. Several arrangements are possible depending on the underlying pathology. All are aimed at protecting the pulmonary circuit and improving systemic perfusion [18,29].
Figure 14.49: First surgical step in the event of hypoplastic left heart. A: palliation according to Norwood (stage 1). This entails: widening of the aortic arch, anastomosis between the proximal PA and aorta, closure of the distal PA and creation of a distal Blalock-Taussig shunt (right subclavianRPA). B: Modified Norwood according to Sano. A conduit between the RV and PA replaces the Blalock-Taussig shunt.
Figure 14.50: Hybrid technique (Giessen procedure) in the event of a hypoplastic left heart and ascending aorta: 1) banding of the right and left pulmonary arteries (green arrows), 2) ductus arteriosus patency maintained by a stent, 3) atrial septostomy (AS arrow) to facilitate mixture of the two circulations. Stenting of the ductus arteriosus and septostomy are achieved by percutaneous catheterisation, while the two bandings are achieved surgically by median sternotomy. These procedures are extracardiac and extrapericardial. Therefore, the single ventricle provides the pulmonary and systemic flow via the ductus arteriosus, while the pulmonary circulation is protected from high pressure by the two bandings.
"Permanent” palliative surgery
If it is not possible to reconstruct two functional chambers due to ventricular hypoplasia, iterative palliative procedures are necessary (univentricular repair). The single functional ventricle is used to ensure high-pressure systemic circulation. The challenge is then to create a set up to ensure pulmonary circulation at a 5 times lower pressure regime. Several arrangements are possible depending on the underlying pathology. All are aimed at protecting the pulmonary circuit and improving systemic perfusion [18,29].
- If the pulmonary flow is insufficient (Qp/Qs < 1), systemic venous blood flow in the PA must be improved. This reconstruction begins with a connection between the SVC and right pulmonary artery, and is called a Glenn shunt. The resulting blood supply is significant since the SVC flow represents half of the central venous return in infants (1/3 in older children). This operation is performed at the age of 3-6 months by right-side thoracotomy without CPB or by sternotomy with CPB. The anastomosis is created between the SVC and the right pulmonary artery (RPA) separated from the PA trunk (unidirectional shunting), or by lateral anastomosis of the SVC near the pulmonary bifurcation (end-to-side anastomosis) maintaining the continuity of the PA (bidirectional shunting). The azygos vein is ligated, the Blalock shunt is interrupted and the PA banding is undone. The Glenn procedure offers the benefit of improving pulmonary flow, lowering its supply pressure, and relieving the pumping ventricle by reducing its volume overload (Figure 14.51).
- The Glenn shunt is secondarily supplemented by the Fontan procedure, which involves diverting all the venous return to the PA by bypassing the RV – the IVC is connected to the right PA by an extracardiac tubular prosthesis or tunnelling inside the RA. A small opening (4 mm) may be created between the conduit and the RA to reduce the risk of venous congestion. The surplus blood running through it is guided directly to the LA via the ASD, which is still present. While this increases systemic left ventricular preload, it does so at the cost of arterial desaturation (Figure 14.52) (see Fontan Physiology below). The Fontan procedure is performed on children aged 2-3 years.
- If the systemic flow is insufficient (Qp/Qs > 1), reconstruction is performed in several stages [29].
- The first stage (stage 1 Norwood or Sano procedure) is performed in the first week of life (see above). However, it does not relieve the single ventricle, which is still required to provide the pulmonary and systemic flow.
- The second stage is performed between the ages of 3 and 6 months. The Ao-PA or RV-PA shunt is ligated and replaced by a bidirectional Glenn anastomosis between the SVC and right PA (Figure 14.51). The venous return, which is dependent on the SVC, therefore bypasses the RV, which reduces its volume overload.
- Reconstruction is completed 1-3 years later by a complete Fontan to ensure that pulmonary flow is compatible with the subject's growth until the patient reaches adulthood (see Fontan Physiology below) (Figure 14.53).
- Hybrid procedures such as the Giessen technique are completed between the ages of 3 and 6 months. A neoaorta is reconstructed, the bandings are undone, and a bidirectional cavopulmonary connection is established (Glenn). The surgical mortality rate is < 5%. This process provides a means of avoiding the harmful effects of CPB in neonates and performing the first two stages of the Norwood procedure in one step. Survival at 10 years is 78% [1,12,36].
- In very complex situations, infants’ survival can only be guaranteed by a neonatal heart transplant. This enables the surgeon to reconstruct the aortic arch with the donor's aorta (see Heart Transplantation).
Figure 14.51: Glenn shunt. Blood from the superior vena cava (SVC) drains into the right pulmonary artery (RPA) via a end-to-side anastomosis (bidirectional Glenn). Blood from the inferior vena cava (IVC) continues to drain into the RA and then into the LA via the ASD.
Figure 14.52: Palliation by Fontan after creating a Glenn shunt (GS). A: extracardiac lateral conduit (yellow). Blood from the inferior vena cava (IVC) drains directly into the right pulmonary artery (RPA). Both vena cavae are ligated at their intrance into the RA. SV: single ventricle. B: tunnelling of a conduit (yellow) inside the RA. It connects the inferior vena cava (IVC) inlet to the superior vena cava (SVC) inlet. The proximal part of the SVC is anastomosed to the RPA. This tunnel is often fenestrated into the RA to prevent overloading of the venous return (acting as a valve in case of high pressure). Blood drains from the RA into the LA via the ASD. In both cases, the pulmonary artery trunk is interrupted.
Figure 14.53: Complete Fontan palliation by a Glenn anastomosis (red arrow) between the superior vena cava (SVC) and the right pulmonary artery (RPA) and by an extracardiac Fontan conduit (FC) (blue arrow) between the inferior vena cava (IVC) and RPA. The proximal trunk of the pulmonary artery (PA) is ligated (pink arrow).
In the postoperative phase of these procedures, young children face several complications.
- Ventricular failure: inotropic support required (dobutamine, epinephrin, milrinone).
- Excessive hypoxaemia: insufficient pulmonary flow is improved by pulmonary vasodilation (NO) and systemic vasoconstriction (but without risking tissue ischaemia and metabolic acidosis).
- Low cardiac output: due to the fall in Qs, it may appear as if pulmonary flow is excessive (Qp/Qs > 1). This is characterised by an increased pulse pressure and a reduced diastolic systemic pressure. It is essential to maintain perfusion pressure with norepinephrin.
- Myocardial ischaemia due to a combination of low diastolic pressure and left ventricular hypertrophy (LVH).
- Arrhythmias.
Ten to twenty years ago, the survival rate at 1 year for these various techniques varied between 75% and 90% [4,16,43]. It is now almost 95%, with 85% 30-year life expectancy [35].
Ventricular hypoplasia |
Tricuspid atresia and hypoplastic RV. The single functional ventricle is the LV.
Hypoplastic left heart and aortic arch: the single functional ventricle is the RV. Characteristics: - Cyanosis due to the mixture of circulations - Volume overload for the single ventricle, which must provide the systemic and pulmonary flow - Impossible to maintain an adequate Qp/Qs ratio Surgical strategy: the single functional ventricle is used to ensure high-pressure systemic circulation, while a special arrangement ensures low-pressure pulmonary circulation. The aim is to ensure a normal Qp/Qs ratio, although SaO2 remains low. - Partial (Glenn) or total (Fontan) cavopulmonary shunt - Staged Norwood or Sano reconstructions - Hybrid procedures (surgery + catheterisation) Children's survival is provided at the cost of non-anatomical reconstruction and non-physiological haemodynamics |
Anaesthesia for univentricular hearts (initial procedures)
The ductus arteriosus of infants with univentricular hearts is kept open by a prostaglandin infusion. This infusion is only discontinued when the CPB is initiated as it is required for ensuring systemic or pulmonary flow. The key points for anaesthetists are as follows [26].
The ductus arteriosus of infants with univentricular hearts is kept open by a prostaglandin infusion. This infusion is only discontinued when the CPB is initiated as it is required for ensuring systemic or pulmonary flow. The key points for anaesthetists are as follows [26].
- The target SaO2 and PaO2 are 70-80% and 40-50 mmHg respectively. Greater oxygenation (SaO2 > 80%) would divert blood flow by raising pulmonary flow and result in a state of systemic shock.
- If pulmonary flow is excessive, the child is ventilated with low FiO2 (0.21-0.3) and hypercapnia (PaCO2 45-50 mmHg). PEEP (3-5 cm H2O) helps impede the pulmonary flow and prevents atelectasis. Adding CO2 (2-4%) to the inspired gases to control PaCO2 without having to hypoventilate the patient with an excessively low tidal volume (TV) also tends to raise SVR. This is mainly performed during CPB [39]. The TV may be kept at 8-10 mL/kg if the respiratory rate is low (< 10 breaths/min).
- Inotropic support (dobutamine, epinephrin-milrinone) is required.
- Normothermia must be strictly maintained, since hypothermia reduces CO2 production and destabilises the Qp/Qs ratio.
Anaesthesia is based on high doses of fentanyl (50-100 mcg/kg), deep sedation (midazolam) to prevent pulmonary hypertensive crises (stress-free anaesthesia), and reduction of systemic VO2 (rocuronium). Mask induction with sevoflurane is generally poorly tolerated. Intravenous induction is preferable. Anaesthesia and controlled ventilation sometimes lower PVR to such an extent that the systemic circulation is compromised.
Following initial surgery, haemodynamics continue as single ventricle circulation. In neonates, PVR may be high post-CPB, requiring inhalation of NO to improve SaO2. Following a Blalock shunt, the diastolic AP is low (15-20 mmHg). This phenomenon is less pronounced after implantation of a Sano-type RV-PA conduit. In the event of ventricular dysfunction and myocardial oedema, closing the thorax may be problematic and therefore the sternum must be left open for 24-48 hours. Constant measurement of cerebral O2 saturation (ScO2) by NIRS (near infrared spectroscopy) is very useful for assessing actual O2 supply to the tissue.
Anaesthesia for secondary palliation
In contrast to the previous situation, the child is older (> 3 months), his pulmonary blood flow is better controlled (Qp:Qs 1.0-1.5:1) and his risk of systemic hypoperfusion is significantly reduced. While mask induction (sevoflurane) is possible, intravenous induction is more stable (fentanyl or sufentanil, etomidate). Maintenance anaesthesia is provided by sevoflurane and fentanyl and in rarer cases by a propofol or midazolam infusion [6]. Children who often undergone several surgical procedures with high doses of fentanyl, sufentanil and midazolam, may have become accustomed to these agents and require higher dosages. Anaesthetic management for the construction of a Glenn anastomosis is focused on several factors [15,26].
- Maintaining normothermia.
- Good analgesia and curarisation (reduction of VO2).
- Protective ventilation by maintaining the lowest possible mean intrathoracic pressure (TV 8-10 mL/kg, rate 10-15 breaths/min, I:E ratio of 1:3 to 1:4).
- Hyperventilation and hypocapnia are beneficial for lowering PVR, but reduce cerebral blood flow. Jugular venous flow accounts for the majority of SVC flow in young children. Consequently, the blood flow from the Glenn shunt to the PA decreases and total pulmonary flow is lowered. Normocapnia is therefore preferable [26].
- Central venous pressure (CVP) is in fact a measure of mean pulmonary arterial pressure (12-18 mmHg; it is possible to measure the transpulmonary gradient (approximately 10 mmHg) with a catheter surgically inserted in the LA.
- SaO2 is 75-80% and Qp:Qs is lower than 1 (0.5-0.7:1).
- The most likely cause of hypoxaemia is low cardiac output. The survival rate is higher if SvO2 is > 30% [17].
- Inotropic stimulation (dobutamine, epinephrin-milrinone)
- Systemic vasodilation (SVR lowered but not excessively), perfusion pressure maintained (norepinephrin).
- Monitoring of lactate – survival is compromised if it increases ≥ 0.75 mmol/L per hour [5].
- Sternum not closed in 50% of cases.
- By placing the child in a sitting position, venous return to the lungs can be improved.
Univentricular heart |
Keep the Qp/Qs ratio as close as possible to 1 (Qp = Qs) without seeking optimal SaO2. Primarily maintain systemic O2 delivery.
Reduce excessive pulmonary flow by hypoventilation and FiO2 of 0.21 (SaO2 75-80%) and increase systemic flow by reducing SVR. Increase the Ω ratio: SaO2 / (SaO2 - SvO2) (Ω > 2.5) Inotropic support (epinephrin-milrinone), deep anaesthesia and curarisation (reduction of VO2) |
Fontan physiology
Several types of Fontan palliation exist. All involve bypassing the tricuspid valve and the RV by diverting venous blood directly to the pulmonary artery. The single ventricle therefore acts as a systemic ventricle, resulting in two circulations in series (Figures 14.52 and 14.53) [33]:
- A single ventricle pumping oxygenated blood into the systemic circulation. Although this SV is not affected by volume overload (cardiac output is low normal), it must provide sufficient kinetic energy for a longer path than the systemic circuit. Its afterload is therefore higher (Figure 14.54). However, it cannot increase its output with exercise, particularly because the anatomical structure of a single ventricle is abnormal.
- A central venous system supplying the pulmonary circulation without assistance from a pump (non-pulsatile flow), and which should be maintained at a high CVP. This venous hypertension induces a systemic stasis and induces a symptomatology of heart failure, even if the systolic function seems to be preserved.
Figure 14.54: Diagram of hydraulic energy supplied by the single ventricle to the Fontan circulation and represented here by intravascular pressure. Normally (solid curves), the LV causes pressure to rise from LA pressure (LAP) to peak systemic arterial pressure (SAP). In line with systemic vascular resistance (SVR), the pressure drops back down to the level of right atrial pressure (RAP). The RV then raises it again to the level of systolic pulmonary arterial pressure (PAPs) after which it falls again, in line with pulmonary vascular resistance (PVR), to the value of left atrial pressure. In the Fontan circulation (dashed curve), the energy supplied by the RV (blue double arrow) is no longer present. It is dependent on the LV, which must pump the blood beyond the systemic venous circuit into the pulmonary circulation. FR: resistance in the Fontan conduit. Red line: left circulation. Blue line: right circulation [26].
Since the SVC drains half of the systemic venous return in young children, the Glenn shunt increases pulmonary flow by half (Qp/Qs: 0.5). However, this proportion decreases with growth and supply to the pulmonary flow becomes insufficient. Around the age of 3 years, the procedure must be completed by diverting all the venous return to the PA. Since its original description in 1971 by F. Fontan and E. Baudet, several changes have been made to the Fontan procedure: direct anastomosis from the RA to the PA (abandoned), lateral tunnel connecting the IVC to the PA via the root of the SVC, tunnelling in the LA between the IVC and SVC and anastomosis of the SVC to the RPA, or prosthetic external conduit from the IVC to the PA (Figures 14.52 and 14.53) [2,21,25].
- The lateral tunnel is constructed by a patch inside the RA creating a cylindrical conduit between the root of the IVC and that of the SVC, which is itself connected to the PA. This IVC→ conduit → SVC→ PA system offers the benefit of growing with the child.
- The direct external IVC → PA conduit offers the best haemodynamics and ensures the lowest RAP as RA simply becomes an extension of the left atrium via the ASD.
- By fenestrating the Fontan conduit into the atrium (approximately 4 mm in diameter), it is possible to limit the risk of excessive pressure in the central venous circuit and maintain the preload of the systemic ventricle in the event of an increase in PVR and a reduction in pulmonary flow. However, this comes at the cost of some arterial desaturation (85-90%) [8]. This fenestration may be performed percutaneously at a later stage or occluded by catheterisation.
- The RA-PA anastomosis results in significant dilation of the RA, which increases the risk of arrhythmia and thrombosis. It is no longer practised.
- Only the coronary sinus and any flow from a fenestration leak into the RA. After a Fontan procedure, Qp/Qs is 0.9 - 1.0.
The operation is performed from the age of 18-24 months, but preferably between 2 and 4 years [33]. The surgical mortality rate of the Fontan procedure is currently < 5% (ideally 1%). Survival at 10 years and 30 years is 90-95% and 60-85% respectively [9,19,31,35,40]. The survival rate is higher in case of hypoplastic RV, since the single systemic ventricle in such instances is an anatomically left ventricle.
The haemodynamics of the Fontan circulation is characterised by an entirely passive pulmonary flow, whose flow rate is solely dependent on central venous pressure. The CVP must therefore be kept high (≥ 15 mmHg). Certain conditions are required for a Fontan procedure to work [13].
- An adequate pulmonary vascular bed;
- Low pulmonary vascular resistance (PVR) (< 3 Wood units/m2);
- Low LAP (≥ 8 mmHg);
- A competent atrioventricular valve;
- A sinus rhythm;
- A systemic ventricle with good systolic and diastolic function (LVEDP < 12 mmHg).
A minimum transpulmonary gradient of 6-8 mmHg is required to ensure the flow between the RA and LA [7]. Any increase in PVR (hypoxia, atelectasis, pneumonia), intrathoracic pressure (Valsalva, IPPV) or LAP (left-sided failure, atrioventricular valve insufficiency, non-sinus rhythm) dramatically reduces pulmonary flow, thereby also reducing systemic ventricle preload. The child becomes cyanotic, hypotensive and acidotic. Contraindications to the Fontan procedure are: PVR > 4 Wood IU, pulmonary hypoplasia, ventricle EF < 0.35, and diastolic dysfunction (LVEDP > 20 mmHg).
The flow velocity in a Fontan conduit is approximately 0.2 – 0.5 m/s, same as in the great central veins. In the absence of a ventricular pump, its blood flow is pulseless. It oscillates during the cardiac cycle with a systolic component and a diastolic component. The loss of pulsatility reduces the production of NO as this is linked to the cyclic wall stress on the pulmonary vascular wall. Unfortunately, this phenomenon contributes to an increase in PVR [23]. High velocity (> 1.5 m/s) indicates stenosis, while a loss of oscillation suggests obstruction [10]. Mean pulmonary flow is largely dependent on the increase in flow occurring at each inspiration due to the suction effect of subatmospheric intrathoracic pressure. In contrast, during IPPV, the flow is reduced or even interrupted with each ventilator inspiration (Figure 14.55). Ideally, these children should therefore be kept in spontaneous ventilation.
The Fontan circulation eliminates the mixture of venous and arterialised blood. Arterial oxygen saturation is normally 90-95% at room air. A drop of saturation below 90% indicates insufficient pulmonary flow. It also eliminates volume overload for the single ventricle, since two circulations are reconstructed in series. However, in the absence of a pulmonary ventricle, the single ventricle must supply the energy required to maintain sufficient venous pressure.
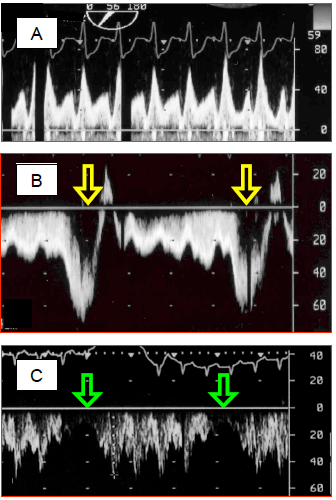
Figure 14.55: Spectral Doppler recordings of flow in a Fontan circuit. A: pulmonary flow in a Fontan circulation – the flow is of a central venous rather than arterial type, with two clearly separated systolic and diastolic components. Vmax is normal for a central vein (< 0.8 m/s). B: during spontaneous respiration, the flow accelerates considerably during inspiration, when intrathoracic pressure falls below atmospheric pressure. This provides valuable assistance for the pulmonary flow. C: in contrast, with IPPV, the flow is interrupted during each positive-pressure inspiration of the ventilator. In B and C, flow is recorded through the transgastric route, which explains why the flow is below the baseline (it moves away from the transducer when flowing from the IVC to the PA).
Although successful in many respects, the Fontan system does not guarantee long-term normal survival: 47% of patients experience complications within 15 years [35]. Failure is manifested by dyspnoea, cyanosis, fluid retention, palpitations and syncopes. Multiple causes can be identified [8,29,33,34].
The flow velocity in a Fontan conduit is approximately 0.2 – 0.5 m/s, same as in the great central veins. In the absence of a ventricular pump, its blood flow is pulseless. It oscillates during the cardiac cycle with a systolic component and a diastolic component. The loss of pulsatility reduces the production of NO as this is linked to the cyclic wall stress on the pulmonary vascular wall. Unfortunately, this phenomenon contributes to an increase in PVR [23]. High velocity (> 1.5 m/s) indicates stenosis, while a loss of oscillation suggests obstruction [10]. Mean pulmonary flow is largely dependent on the increase in flow occurring at each inspiration due to the suction effect of subatmospheric intrathoracic pressure. In contrast, during IPPV, the flow is reduced or even interrupted with each ventilator inspiration (Figure 14.55). Ideally, these children should therefore be kept in spontaneous ventilation.
The Fontan circulation eliminates the mixture of venous and arterialised blood. Arterial oxygen saturation is normally 90-95% at room air. A drop of saturation below 90% indicates insufficient pulmonary flow. It also eliminates volume overload for the single ventricle, since two circulations are reconstructed in series. However, in the absence of a pulmonary ventricle, the single ventricle must supply the energy required to maintain sufficient venous pressure.
Figure 14.55: Spectral Doppler recordings of flow in a Fontan circuit. A: pulmonary flow in a Fontan circulation – the flow is of a central venous rather than arterial type, with two clearly separated systolic and diastolic components. Vmax is normal for a central vein (< 0.8 m/s). B: during spontaneous respiration, the flow accelerates considerably during inspiration, when intrathoracic pressure falls below atmospheric pressure. This provides valuable assistance for the pulmonary flow. C: in contrast, with IPPV, the flow is interrupted during each positive-pressure inspiration of the ventilator. In B and C, flow is recorded through the transgastric route, which explains why the flow is below the baseline (it moves away from the transducer when flowing from the IVC to the PA).
Although successful in many respects, the Fontan system does not guarantee long-term normal survival: 47% of patients experience complications within 15 years [35]. Failure is manifested by dyspnoea, cyanosis, fluid retention, palpitations and syncopes. Multiple causes can be identified [8,29,33,34].
- Atrial tachyarrhythmias are common (incidence 10% after 10 years and 45% after 25 years) and poorly tolerated as they increase the pulmonary circuit’s downstream pressure. They are often associated with atrial dilation. The prevalence of ventricular tachycardia is 2-12% [22,22,38].
- Since normal saturation at room air is only 90-95%, the slightest fall in pulmonary flow or the slightest increase in O2 demand causes hypoxaemia SaO2 < 90%). Thiy cyanosis leads to polycythemia.
- The single ventricle must provide the systemic circulation and supply the energy required to maintain sufficient central venous pressure for the pulmonary circulation. If its function deteriorates, its diastolic pressure increases, which raises the downstream pressure of the pulmonary vascular bed and immediately slows pulmonary flow. Ventricular failure is evident in 50% of patients above the age of 20 years [28]. Since the preload reserve is low, flow mainly increases through tachycardia. Ventricular failure occurs earlier and more frequently if the single ventricle exhibits the morphology of a right ventricle.
- Since CVP must remain high to ensure pulmonary blood flow, systemic venous stasis is common. Twenty-eight percent of children present with fluid retention, hepatomegaly, ascites, and protein-losing enteropathy [9]. These side effects, which may be limited by fenestrating the Fontan conduit, are associated with protein loss and long-term liver dysfunction, which may result in cardiac cirrhosis. They culminate in hypoproteinaemia and significant changes in clotting factor synthesis entailing a significant risk of haemorrhage [41]. In the long term, the mean incidence of thromboembolism is 25% and that of renal dysfunction is 50% [42].
- The advent of a regurgitation through the AV valve, which is more frequent when the valve is tricuspid, compromises severely the Fontan circulation, because it induces a volume overload for the ventricle and an increase in the downstream pressure for the pulmopnary flow.
- Loss of pulmonary flow pulsatility tends to remodel vessels and increase PVR. Phosphodiesterase-5 inhibitors (sildenafil, tadalafil) and endothelin receptor antagonists (bosentan, ambrisentan) improve patients’ ability for exertion [14]. It is essential to continue administering these drugs perioperatively.
When the ventricular failure becomes irreversible, the only conceivable therapy is a heart transplant. The mechanical assist devices are not performing well because none of these devices can provide a high output with a low pressure adapted to the cavo-pulmonary circulation [33]. The one-year survival rate after transplantation is 75-85% among children [37].
Anaesthesia for a Fontan procedure
Prior to the procedure, SaO2 is 75-80%. Mask induction is difficult as coughing or a Valsalva manoeuvre impede the pulmonary circulation. Pulmonary flow is slowed because the Qp:Qs ratio is 0.5-0.7. Intravenous induction is preferable (fentanyl or sufentanil, midazolam). Post-CPB, mean intrathoracic pressure must remain minimal (tidal volume 8 mL/kg, rate 10-15 breaths/min, I:E ratio 1:3-4). CVP is used to assess PAPmean (15-18 mmHg). It is possible to measure LAP and the transpulmonary gradient (approximately 10 mmHg) with a catheter surgically inserted in the LA. Fontan haemodynamics are excessively sensitive to hypovolaemia, and in particular to the reduction of central venous return prompted by agents such as propofol [26].
Anaesthesia in cases where Fontan circulation is present
Anaesthetists should encourage pulmonary flow, which is passive, and maintain systemic ventricle function [8,20,22]. The reduction in cardiac output is largely due to reduced preload (hypovolaemia), raised PVR, ventricular dysfunction, or arrhythmias [44].
Anaesthesia for a Fontan procedure
Prior to the procedure, SaO2 is 75-80%. Mask induction is difficult as coughing or a Valsalva manoeuvre impede the pulmonary circulation. Pulmonary flow is slowed because the Qp:Qs ratio is 0.5-0.7. Intravenous induction is preferable (fentanyl or sufentanil, midazolam). Post-CPB, mean intrathoracic pressure must remain minimal (tidal volume 8 mL/kg, rate 10-15 breaths/min, I:E ratio 1:3-4). CVP is used to assess PAPmean (15-18 mmHg). It is possible to measure LAP and the transpulmonary gradient (approximately 10 mmHg) with a catheter surgically inserted in the LA. Fontan haemodynamics are excessively sensitive to hypovolaemia, and in particular to the reduction of central venous return prompted by agents such as propofol [26].
Anaesthesia in cases where Fontan circulation is present
Anaesthetists should encourage pulmonary flow, which is passive, and maintain systemic ventricle function [8,20,22]. The reduction in cardiac output is largely due to reduced preload (hypovolaemia), raised PVR, ventricular dysfunction, or arrhythmias [44].
- PVR must remain low in order to maintain flow through the lungs. Acidosis, hypoxaemia and hypercapnia must therefore be avoided. It is essential to maintain hypocapnia (PaCO2 35 mmHg, pH 7.45) through moderate hyperventilation. Fi O2 is adjusted to 0.5-0.8. Bicarbonate or TRIS may be required to return pH to normal levels.
- PVR may be reduced by hypobaric hyperventilation, inhalation of NO (10-30 ppm), prostaglandins (inhaled or IV), sildenafil (IV) or magnesium (IV) and by an inodilator such as milrinone.
- Inspiration during spontaneous respiration draws the blood into the lungs, which increases the pulmonary blood flow. In contrast, controlled ventilation reduces or interrupts the pulmonary flow at each ventilator inspiration (see Figure 14.55). Therefore, when possible, these patients should be kept in spontaneous ventilation, provided that they are not hypoventilating. If IPPV is required, the mean ventilation pressure should be kept as low as possible. The best approach to ventilation is to prevent hypoxia, hypercapnia and atelectasis, and keep mean intrathoracic pressure at minimal levels (protective ventilation, I:E ratio of 1:3-4, no PEEP). The adequacy of blood flow in the Fontan circuit may be monitored by TEE.
- Liberal use of NO limits the risk of increasing PVR and reducing pulmonary blood flow.
- Hypovolaemia is extremely poorly tolerated since the reduction in preload instantly reduces the pulmonary flow (fall in CVP). Tachycardia is the sole means of increasing cardiac output. Fluid filling should be performed during induction.
- Since it is impossible to increase pulmonary blood flow in the event of anaemia, the transfusion threshold is high (Hb ≥ 100 g/L).
- Ventricular function is generally limited and in most cases requires inotropic stimulation. Milrinone and epinephrin is the most effective combination. Any deterioration in systemic ventricular function increases the pressure in the LA and reduces the transpulmonary gradient, thereby also reducing pulmonary flow. Anaesthetic agents should be chosen on the basis of this poor ventricular performance.
- The central venous line (CVP) actually measures pulmonary arterial driving pressure (PAP). It is very useful to monitor this measurement, but the risk of thrombosis is high. It is strongly advised to refrain from catheterising internal jugular veins or subclavian veins in children with a Glenn shunt. It is less dangerous to measure venous pressure in the external jugular vein (short Venflon™ type catheter) or in the femoral vein (10-15 cm catheter). TEE offers a less invasive and safer means of monitoring preload. CVP must be kept at approximately 15 mmHg.
- Recommended monitoring: SpO2, ECG, arterial catheter, venous pressure, ETCO2, ScO2, TEE. TEE is used to determine whether the filling of the systemic ventricle is adequate, irrespective of the CVP value. At the end of the procedure, a transthoracic catheter in the LA is used to calculate the transpulmonary gradient (CVP – LAP).
- Several options are available for induction.
- Ketamine (1-2 mg/kg IV) ensures haemodynamic stability due to its central sympathetic stimulation. However, it has a direct cardiodepressant effect on the myocardium. In children, it does not raise PVR, provided that ventilation and oxygenation are maintained.
- Midazolam (0.1 mg/kg) significantly lowers preload, which reduces pulmonary flow and deepens cyanosis.
- Propofol excessively reduces preload and is therefore not a good option.
- In highly unstable cases, etomidate (0.3 mg/kg) is the safest agent.
- Fentanyl (10-25 mcg/kg) and sufentanil (0.5-2.5 mcg/kg) tend to prevent PVR from rising and enable doses of induction agents to be reduced, thereby limiting their haemodynamic effects.
- Administering sevoflurane by mask is an appropriate technique in the absence of a venous line.
- On induction, administration of a crystalloid bolus (10-15 mL/kg) is recommended to prevent any reduction in preload associated with anaesthesia [26].
- If inotropic support is required, an epinephrin-milrinone combination is the preferred choice.
- In the postoperative phase, spontaneous breathing should be resumed as quickly as possible. The patient is kept in a sitting position.
Fontan circulation |
Characteristics:
- Bypassing the RV by diverting the SVC + IVC directly to the PA - Reduced pulmonary flow (Qp ↓); driving pressure: CVP - Pulmonary perfusion pressure: CVP - LAP (ΔP min: 8-10 mmHg) - Normal SpO2: 90-95% Functional conditions: - CVP ≥ 15 mmHg - LAP ≤ 8 mmHg - Low PVR - Low intrathoracic P - Normal LV and atrioventricular valve function - No arrhythmia Sequelae: arrhythmias, venous stasis, ascites, thrombosis, LV dysfunction Anaesthesia recommendations: - Spontaneous ventilation if possible – avoid any hypoventilation - Ventilation: FiO2 ↑, hypocapnia, alkalosis, low intrathoracic Pmean, no PEEP, NO - Hypovolaemia poorly tolerated (reduction in pulmonary flow due to lower CVP) |
© BETTEX D, BOEGLI Y, CHASSOT PG, June 2008, last update February 2020
References
- AKINTURK H, MICHEL-BEHNKE I, VALESKE K, et al. Hybrid transcatheter-surgical palliation : basis for univentricular or biventricular repair : the Giessen experience. Pediatr Cardiol 2007 ; 28 :79-87
- BALAJI S, GEWILLIG M, BULL C, et al. Arrhythmias after Fontan procedure: comparison of total cavopulmonary connection to atriopulmonary connection. Circulation 1991; 84(Suppl III):162-7
- BARNEA O, SANTAMORE WP, ROSSI A, et al. Estimation of oxygen delivery in newborns with a univentricular circulation, Circulation 1998; 98:1407-13
- CALDARONE CA, BENSON L, HOLTBY H, et al. Initial experience with hybrid palliation for neonates with single-ventricle physiology. Ann Thorac Surg 2007 ; 84 :1294-300
- CHARPIE JR, DEKEON MK, GOLDBERG CS, et al. Serial blood lactate measurements predict early outcome after neonatal repair or palliation for complex congenital heart disease J Thorac Cardiovasc Surg 2000; 120:73-80
- CHRISTENSEN RE, GHOLAMI AS, REYNOLDS PI, et al. Anaesthetic management and outcomes after noncardiac surgery in patients with hypoplastic left heart syndrome: a retrospective review. Eur J Anaesthesiol 2012; 29:425-30
- DISSESSA TG, CHILD JS, PERLOFF JK, et al. Systemic venous and pulmonary arterial flow patterns after Fontan’s procedure for tricuspid atresia or single ventricle. Circulation 1984; 70:898-902
- EAGLE SS, DAVES SM. The adult with Fontan physiology: systematic approach to perioperative management for noncardiac surgery. J Cardiothorac Vasc Anesth 2011; 25:320-34
- FONTAN F, KIRKLIN JW, FERNANDEZ G, et al. Outcome after a "perfect" Fontan operation. Circulation 1990; 81:1520-36
- FYFE DA, KLINE CH, SADE RM, GREENE CA, GILETTE PC. The utility of transesophageal echocardiography during and after Fontan operations in small children. Am Heart J 1991; 122:1403-15
- GACA JA, DOUGLAS WI, BARNES SD. Anesthetic implications of the Fontan procedure for single ventricle physiology. Sem Cardiothorac Vasc Anesth 2001 ; 5 :31-9
- GALANTOWICZ M, YATES AR. Improved outcomes with the comprehensive stage 2 procedure after an initial hybrid sate 1. J Thorac Cardiovasc Surg 2016; 151:424-9
- GENTLES TL, MAYER JE Jr, GAUVREAU K, et al. Fontan operation in five hundred consecutive patients: factors influencing early and late outcome. J Thorac Cardiovasc Surg 1997; 114:376-91
- GOLDBERG DJ, FRENCH B, McBRIDE MG, et al. Impact of oral sildenafil on exercise performance in children and young adults after the fontan operation: a randomized, double-blind, placebo-controlled, crossover trial. Circulation 2011; 123:1185-93
- GOTTLIEB EA, ANDROPOULOS D. Anesthesia for the patient with congenital heart disease presenting for noncardiac surgery. Curr Opin Anesthesiol 2013; 26:318-26
- HEHIR DA, DOMINGUEZ TE, BALLWEG JA, et al. Risk factors for interstage death after stage I reconstruction of hypoplastic left heart syndrome and variants. J Thorac Cardiovasc Surg 2008 ; 136 :94-9
- HOFFMAN GM, GHANAYEM NS, KAMPINE JM, et al. Venous saturation and the anaerobic threshold in neonates after Norwood procedure for hypoplastic left heart syndrome. Ann Thorac Surg 2000; 70:1515-20
- HONJO O, Van ARSDELL GS. Cardiovascular procedures : surgical considerations. In : BISSONNETTE B, edit. Pediatric anesthesia. Basic principles, State of the art, Future. Shelton (CO): People’s Medical Publishing House (USA), 2011, 1589-608
- HOSEIN RB, CLARK AJ, McGUIRK SP, et al. Factors influencing early and late outcome following the Fontan procedure in the current era. Eur J Cardiothorac Surg 2007; 31:344-53
- HOSKING MP, BEYNEN FM. The modified Fontan procedure: physiologic and anesthetic implications. J Cardiothorac Vasc Anesth 1992; 6:465-7
- KUMAR SP, RUBENSTEIN CS, SIMSIC JM, et al. Lateral tunnel versus extracardiac conduit Fontan procedure: a concurrent comparison. Ann Thorac Surg 2003; 76:1389-97¨
- LASA JJ, GLATZ AC, DAGA A; et al. Prevalence of arrhythmias late after the Fontan operation. Am J Cardiol 2014; 113:184-8
- LEYVI G, WASNICK JD. Single-ventricle patient: pathophysiology and anesthetic management. J Cardiothorac Vasc Anesth 2010; 24:121-30
- LI J, ZHANG G, McCRINDLE BW, et al. Profiles of hemodynamics and oxygen transport derived by using continuous measured oxygen consumption after the Norwood procedure. J Thorac Cardiovasc Surg 2007; 133:441-8
- MARCELETTI CF, HANLEY FL, MAVROUDIS C, et al. Revisions of previous Fontan connections to total extracardiac cavopulmonary anastomosis: A multicenter experience. J Thorac Cardiovasc Surg 2000; 119:340-6
- NASR VG, DINARDO JA. The pediatric cardiac anesthesia handbook. Oxford: Wiley-Blackwell, 2017, 161-85
- NICOLSON SC, STEVEN JM. Anesthesia for the patient with a single ventricle. In : ANDROPOULOS DA, et al, eds. Anesthesia for congenital heart disease. Oxford: Blackwell-Futura, 2005, 356-72
- NOROSI K, WESSEL A, ALPERS V, et al. Incidence and risk distribution of heart failure in adolescents and adults with congenital heart disease after cardiac surgery. Am J Cradiol 2006; 97:1238-43
- OHYE RG, SCHRANZ D, D'UDEKEM Y. Current therapy for hypoplastic left heart syndrome and related single ventricle lesions. Circulation 2016; 134:1265-79
- OHYE RG, SLEEPER LA, MAHONY L, et al. Comparison of shunt types in the Norwood procedure for single-ventricle lesions. N Engl J Med 2010 ; 362 :1980-92
- PUNDI KN, JOHNSON JN, DEARANI JA; et al. 40-year follow-up after the Fontan operation: long-term outcomes of 1'052 patients. J Am Coll Cardiol 2015; 66:621-2
- RIORDAN CJ, RANDSBAEK F, STOREY JH, et al. Inotropes in the hypoplastic left heart syndrome : effects in an animal model. Ann Thorac Surg 1996 ; 62 :83-90
- RYCHIK J, ATZ AM, CELERMAJER D, et al. Evaluation and management of the child and adult with Fontan circulation. A Scientific Statement of the American Heart Association. Circulation 2019; 140:e234-e284
- RYCHIK J, GOLDBERG DJ. Late consequences of the Fontan operation. Circulation 2014;130:1525-8
- SCHILLING C, DALZIEL K, NUNN R, et al. The Fontan epidemic: population projections from the Australia and New Zealand Fontan Registry. Int J Cardiol 2016; 219:14-9
- SCHRANZ D, BAUER A, REICH B, et al. Fifteen year single-center experience with the "Giessen hybrid" approach for hypoplastic left heart and variants: current strategies and outcomes. Pediatr Cardiol 2015; 36:365-73
- SIMPSON KE, PRUITT E, KIRKLIN JK, et al. Fontan patient survival after pediatric heart transplantation has improved in the current era. Ann Thorac Surg 2017; 103:1315-20
- STAMM C, FRIEHS I, MAYER JE, et al. Long-term results of the lateral tunnel Fontan operation. J Thorac Cardiovasc Surg 2000; 121:28-41
- TABBUTT S, RAMAMOORTHY C, MONTENEGRO LM, et al. Impact of inspired gaz mixtures on preoperative infants with hypoplastic left heart syndrome during controlled ventilation. Circulation 2001 ; 104 :1159-64
- D’UDEKEM Y, IYENGAR AJ, COCHRANE AD, et al. The Fontan procedure: contemporary techniques have improved long-term outcomes. Circulation 2007; 116:157-64
- VAN NIEUWENHUIZEN RC, PETERS M, LUBBERS TJ, ET AL. Abnormalities in liver function and coagulation profile following the Fontan procedure. Heart11999; 82:40-6
- VARMA C, WARR MR, HENDLER AL, et al. Prevalence of "silent" pulmonary emboli in adults after the Fontan operation. J Am Coll Cardiol 2003; 41:2252-8
- WEINSTEIN S, GAYNOR JW, BRIDGES ND, et al. Early survival of infants weighing 2.5 kilograms or less undergoing first-stage reconstruction for hypoplastic left heart syndrome. Circulation 1999; 100:II167-70
- WINDSOR J, TOWNSLEY MM, BRISTON D, et al. Fontan palliation for single-ventricle physiology: perioperative management for noncardiac surgery and analysis of outcomes. J Cardiothorac Vasc Anesth 2017; 31:2296-303